Origin of High Diastereoselectivity in Reactions of Seven-Membered-Ring Enolates
- PMID: 35076978
- PMCID: PMC8940697
- DOI: 10.1002/anie.202114183
Origin of High Diastereoselectivity in Reactions of Seven-Membered-Ring Enolates
Abstract
Unlike many reactions of their six-membered-ring counterparts, the reactions of chiral seven-membered-ring enolates are highly diastereoselective. Diastereoselectivity was observed for a range of substrates, including lactam, lactone, and cyclic ketone derivatives. The stereoselectivity arises from torsional and steric interactions that develop when electrophiles approach the diastereotopic π-faces of the enolates, which are distinguished by subtle differences in the orientation of nearby atoms of the ring.
Keywords: Conformational Analysis; Diastereoselectivity; Enolates; Seven-Membered-Rings; Torsional Strain.
© 2022 Wiley-VCH GmbH.
Figures
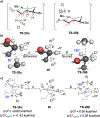
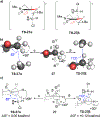
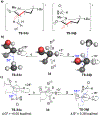
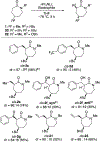
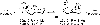
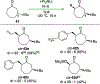
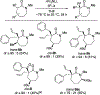
Similar articles
-
Diastereoselective synthesis of five- and seven-membered rings by [2+2+1], [3+2], [3+2+2], and [4+3] carbocyclization reactions of beta-substituted (alkenyl)(methoxy)carbene complexes with methyl ketone lithium enolates.Chemistry. 2005 Aug 19;11(17):4995-5006. doi: 10.1002/chem.200500164. Chemistry. 2005. PMID: 15959861
-
Highly diastereoselective alpha-hydroxylation of Fox chiral auxiliary-based amide enolates with molecular oxygen.Org Lett. 2010 Apr 2;12(7):1496-9. doi: 10.1021/ol100211s. Org Lett. 2010. PMID: 20218638
-
Diastereo- and enantioselective iridium-catalyzed allylation of cyclic ketone enolates: synergetic effect of ligands and barium enolates.J Am Chem Soc. 2014 Nov 12;136(45):15825-8. doi: 10.1021/ja506500u. Epub 2014 Oct 30. J Am Chem Soc. 2014. PMID: 25337972 Free PMC article.
-
[Development of new synthetic reactions featuring tandem carbon-carbon bond formation].Yakugaku Zasshi. 2007 Sep;127(9):1399-418. doi: 10.1248/yakushi.127.1399. Yakugaku Zasshi. 2007. PMID: 17827921 Review. Japanese.
-
Generation and Reactivity of C(1)-Ammonium Enolates by Using Isothiourea Catalysis.Chemistry. 2021 Jan 21;27(5):1533-1555. doi: 10.1002/chem.202002059. Epub 2020 Nov 10. Chemistry. 2021. PMID: 32557875 Free PMC article. Review.
Cited by
-
Acetal Substitution Reactions: Stereoelectronic Effects, Conformational Analysis, Reactivity vs. Selectivity, and Neighboring-Group Participation.Synlett. 2024 Sep;35(15):1763-1787. doi: 10.1055/s-0042-1751541. Epub 2024 Jan 16. Synlett. 2024. PMID: 39502501
-
Diastereoselective Alkylations of Chiral Tetrazolo[1,5-a]azepines via Heterobenzylic Anion Intermediates.Org Lett. 2022 Sep 23;24(37):6722-6727. doi: 10.1021/acs.orglett.2c02445. Epub 2022 Sep 12. Org Lett. 2022. PMID: 36095147 Free PMC article.
References
-
- Evans DA, in Asymmetric Synthesis, Vol. 3 (Ed.: Morrison JD), Academic Press, New York, 1984, p. 1.
-
- Mulzer J, Kerkmann T, J. Am. Chem. Soc 1980, 102, 3620–3622;
- Lee SH, Bull. Korean Chem. Soc 2013, 34, 121–127;
- Baldwin JE, Adlington RM, Gollins DW, Schofield CJ, Tetrahedron Lett 1990, 46, 4733–4748;
- Meiries S, Marquez R, J. Org. Chem 2008, 73, 5015–5021; - PubMed
- Lin YC, Ribaucourt A, Moazami Y, Pierce JG, J. Am. Chem. Soc 2020, 142, 9850–9857. - PMC - PubMed
-
- Dostie S, Prévost M, Mochirian P, Tanveer K, Andrella N, Rostami A, Tambutet G, Guindon Y, J. Org. Chem 2016, 81, 10769–10790; - PubMed
- Miyaoka H, Hara Y, Shinohara I, Kurokawa T, Yamada Y, Tetrahedron Lett 2005, 46, 7945–7949;
- Soulieman A, Gouault N, Roisnel T, Justaud F, Boustie J, Grée R, Hachem A, Synlett 2019, 30, 2258–2262;
- Johnson TA, Jang DO, Slafer BW, Curtis MD, Beak P, J. Am. Chem. Soc 2002, 124, 11689–11698; - PubMed
- McAtee JJ, Schinazi RF, Liotta DC, J. Org. Chem 1998, 63, 2161–2167;
- Wångsell F, Gustafsson K, Kvarnström I, Borkakoti N, Edlund M, Jansson K, Lindberg J, Hallberg A, Rosenquist Å, Samuelsson B, Eur. J. Med. Chem 2010, 45, 870–882; - PubMed
- Reddy CR, Dharmapuri G, Rao NN, Org. Lett 2009, 11, 5730–5733; - PubMed
- Konas DW, Coward JK, J. Org. Chem 2001, 66, 8831–8842. - PubMed
-
- Morgans DJ, Tetrahedron Lett 1981, 22, 3721–3724;
- White JD, Somers TC, Reddy GN, J. Am. Chem. Soc 1986, 108, 5353–5354;
- Pinyarat W, Mori K, Biosci. Biotech. Biochem 2014, 57, 419–421;
- Abels F, Lindemann C, Schneider C, Chem. Eur. J 2014, 20, 1964–1979; - PubMed
- Coe D, Drysdale M, Philps O, West R, Young DW, J. Chem. Soc., Perkin Trans 1 2002, 2459–2472.
Publication types
MeSH terms
Substances
Grants and funding
LinkOut - more resources
Full Text Sources
Research Materials
Miscellaneous