Ovotransferrin Supplementation Improves the Iron Absorption: An In Vitro Gastro-Intestinal Model
- PMID: 34829772
- PMCID: PMC8615417
- DOI: 10.3390/biomedicines9111543
Ovotransferrin Supplementation Improves the Iron Absorption: An In Vitro Gastro-Intestinal Model
Abstract
Transferrins constitute the most important iron regulation system in vertebrates and some invertebrates. Soluble transferrins, such as bovine lactoferrin and hen egg white ovotransferrin, are glycoproteins with a very similar structure with lobes that complex with iron. In this in vitro study, a comparison of bovine lactoferrin and ovotransferrin was undertaken to confirm the comparability of biological effects. An in vitro gastric barrier model using gastric epithelial cells GTL-16 and an in vitro intestinal barrier model using CaCo-2 cells was employed to evaluate iron absorption and barrier integrity. An analysis of the molecular pathways involving DMT-1 (divalent metal transporter 1), ferritin and ferroportin was also carried out. These in vitro data demonstrate the activity of both 15% saturated and 100% saturated ovotransferrin on the iron regulation system. Compared with the commercial bovine lactoferrin, both 15% saturated and 100% saturated ovotransferrin were found to act in a more physiological manner. Based on these data, it is possible to hypothesise that ovotransferrin may be an excellent candidate for iron supplementation in humans; in particular, 15% saturated ovotransferrin is the overall best performing product. In vivo studies should be performed to confirm this in vitro data.
Keywords: bovine lactoferrin; gastro-intestinal barrier; iron absorption; iron metabolism; iron transportation; ovotransferrin.
Conflict of interest statement
The authors declare that they have no competing interests. F.U. and C.M. are co-founders of the Noivita Srls start-up at the University of Eastern Piedmont. P.G. is funded by BIOSEUTICA B.V. but had no role in the design of the study, analyses, or interpretation of data or in the decision to publish the results.
Figures
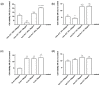
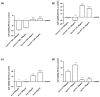
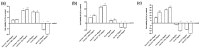
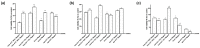
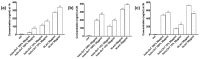
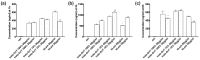
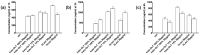
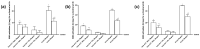
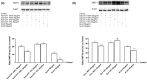
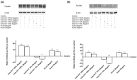
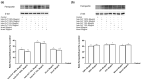
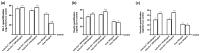
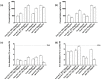
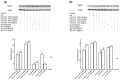
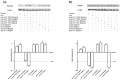
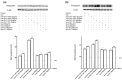
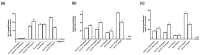
Similar articles
-
Metal ligand-induced alterations in the surface structures of lactoferrin and transferrin probed by interaction with immobilized copper(II) ions.J Chromatogr. 1991 Jan 4;536(1-2):1-15. doi: 10.1016/s0021-9673(01)89232-3. J Chromatogr. 1991. PMID: 2050759
-
Physiological roles of ovotransferrin.Biochim Biophys Acta. 2012 Mar;1820(3):218-25. doi: 10.1016/j.bbagen.2011.08.004. Epub 2011 Aug 10. Biochim Biophys Acta. 2012. PMID: 21854833 Review.
-
The Iron Binding Ability Maps the Fate of Food-Derived Transferrins: A Review.J Agric Food Chem. 2024 Aug 14;72(32):17771-17781. doi: 10.1021/acs.jafc.4c04827. Epub 2024 Aug 1. J Agric Food Chem. 2024. PMID: 39087686 Review.
-
Antiviral activity of ovotransferrin discloses an evolutionary strategy for the defensive activities of lactoferrin.Biochem Cell Biol. 2002;80(1):125-30. doi: 10.1139/o01-208. Biochem Cell Biol. 2002. PMID: 11908636
-
Crystal structure of diferric hen ovotransferrin at 2.4 A resolution.J Mol Biol. 1995 Nov 24;254(2):196-207. doi: 10.1006/jmbi.1995.0611. J Mol Biol. 1995. PMID: 7490743
Cited by
-
The Combined Effect of Green Tea, Saffron, Resveratrol, and Citicoline against Neurodegeneration Induced by Oxidative Stress in an In Vitro Model of Cognitive Decline.Oxid Med Cell Longev. 2024 Oct 1;2024:7465045. doi: 10.1155/2024/7465045. eCollection 2024. Oxid Med Cell Longev. 2024. PMID: 39380915 Free PMC article.
-
Palmitoylethanolamide as a Supplement: The Importance of Dose-Dependent Effects for Improving Nervous Tissue Health in an In Vitro Model.Int J Mol Sci. 2024 Aug 21;25(16):9079. doi: 10.3390/ijms25169079. Int J Mol Sci. 2024. PMID: 39201765 Free PMC article.
-
Design of Mixed Medicinal Plants, Rich in Polyphenols, Vitamins B, and Palmitoylethanolamide-Based Supplement to Help Reduce Nerve Pain: A Preclinical Study.Int J Mol Sci. 2024 Apr 27;25(9):4790. doi: 10.3390/ijms25094790. Int J Mol Sci. 2024. PMID: 38732008 Free PMC article.
-
The Combined Antioxidant Effects of N-Acetylcysteine, Vitamin D3, and Glutathione from the Intestinal-Neuronal In Vitro Model.Foods. 2024 Mar 1;13(5):774. doi: 10.3390/foods13050774. Foods. 2024. PMID: 38472887 Free PMC article.
-
Preliminary study on comparison of egg extraction methods for development of fetal bovine serum substitutes in cultured meat.Food Chem X. 2024 Feb 8;21:101202. doi: 10.1016/j.fochx.2024.101202. eCollection 2024 Mar 30. Food Chem X. 2024. PMID: 38434697 Free PMC article.
References
-
- Bresson J.L., Burlingame B., Dean T., Fairweather-Tait S., Heinonen M., Hirsch-Ernst K.I., Mangelsdorf I., McArdle H., Naska A., Neuhauser-Berthold M., et al. Scientific Opinion on Dietary Reference Values for iron. EFSA J. 2015;13 doi: 10.2903/j.efsa.2015.4254. - DOI
-
- Goroll A.H., Mulley A.G. Primary Care Medicine. Volume 82. Ergodebooks; Houston, TX, USA: 2009. Office evaluation and management of the adult patient; pp. 607–608.
-
- WHO . INT WHO. Report: Priorities in the Assessment of Vitamin A and Iron Status in Populations, Panama City, Panama, 15–17 September 2010. World Health Organization; Geneva, Switzerland: 2012.
LinkOut - more resources
Full Text Sources
Other Literature Sources