Biophysics of Chromatin Remodeling
- PMID: 33395550
- PMCID: PMC8428145
- DOI: 10.1146/annurev-biophys-082520-080201
Biophysics of Chromatin Remodeling
Abstract
As primary carriers of epigenetic information and gatekeepers of genomic DNA, nucleosomes are essential for proper growth and development of all eukaryotic cells. Although they are intrinsically dynamic, nucleosomes are actively reorganized by ATP-dependent chromatin remodelers. Chromatin remodelers contain helicase-like ATPase motor domains that can translocate along DNA, and a long-standing question in the field is how this activity is used to reposition or slide nucleosomes. In addition to ratcheting along DNA like their helicase ancestors, remodeler ATPases appear to dictate specific alternating geometries of the DNA duplex, providing an unexpected means for moving DNA past the histone core. Emerging evidence supports twist-based mechanisms for ATP-driven repositioning of nucleosomes along DNA. In this review, we discuss core experimental findings and ideas that have shaped the view of how nucleosome sliding may be achieved.
Keywords: SF2 superfamily; Snf2-type ATPase motor; bulge/loop propagation; histone; nucleosome; twist defect.
Figures
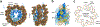
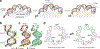
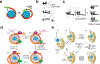
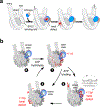
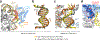
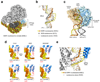
Similar articles
-
Mechanisms of ATP-dependent nucleosome sliding.Curr Opin Struct Biol. 2010 Feb;20(1):73-81. doi: 10.1016/j.sbi.2009.12.002. Epub 2010 Jan 8. Curr Opin Struct Biol. 2010. PMID: 20060707 Free PMC article. Review.
-
Chromatin remodelers couple inchworm motion with twist-defect formation to slide nucleosomal DNA.PLoS Comput Biol. 2018 Nov 5;14(11):e1006512. doi: 10.1371/journal.pcbi.1006512. eCollection 2018 Nov. PLoS Comput Biol. 2018. PMID: 30395604 Free PMC article.
-
A twist defect mechanism for ATP-dependent translocation of nucleosomal DNA.Elife. 2018 May 29;7:e34100. doi: 10.7554/eLife.34100. Elife. 2018. PMID: 29809147 Free PMC article.
-
Interdomain Communication of the Chd1 Chromatin Remodeler across the DNA Gyres of the Nucleosome.Mol Cell. 2017 Feb 2;65(3):447-459.e6. doi: 10.1016/j.molcel.2016.12.011. Epub 2017 Jan 19. Mol Cell. 2017. PMID: 28111016 Free PMC article.
-
Targeting chromatin remodelers: signals and search mechanisms.Biochim Biophys Acta. 2011 Sep;1809(9):497-508. doi: 10.1016/j.bbagrm.2011.06.005. Epub 2011 Jun 16. Biochim Biophys Acta. 2011. PMID: 21704204 Review.
Cited by
-
The histone demethylase JMJ27 acts during the UV-induced modulation of H3K9me2 landscape and facilitates photodamage repair.Nat Plants. 2024 Nov;10(11):1698-1709. doi: 10.1038/s41477-024-01814-9. Epub 2024 Oct 4. Nat Plants. 2024. PMID: 39367258
-
The spread of chemical biology into chromatin.J Biol Chem. 2024 Sep 12;300(11):107776. doi: 10.1016/j.jbc.2024.107776. Online ahead of print. J Biol Chem. 2024. PMID: 39276931 Free PMC article. Review.
-
Muscle-specific pyruvate kinase isoforms, PKM1 and PKM2, regulate mammalian SWI/SNF proteins and histone 3 phosphorylation during myoblast differentiation.FASEB J. 2024 Jun 15;38(11):e23702. doi: 10.1096/fj.202400784R. FASEB J. 2024. PMID: 38837439
-
The nucleic acid binding protein SFPQ represses EBV lytic reactivation by promoting histone H1 expression.Nat Commun. 2024 May 16;15(1):4156. doi: 10.1038/s41467-024-48333-x. Nat Commun. 2024. PMID: 38755141 Free PMC article.
-
Widespread impact of nucleosome remodelers on transcription at cis-regulatory elements.bioRxiv [Preprint]. 2024 Apr 15:2024.04.12.589208. doi: 10.1101/2024.04.12.589208. bioRxiv. 2024. PMID: 38659863 Free PMC article. Preprint.
References
-
- Aalfs JD, Narlikar GJ, Kingston RE. 2001. Functional differences between the human ATP-dependent nucleosome remodeling proteins BRG1 and SNF2H. J. Biol. Chem. 276:34270–8 - PubMed
-
- Allis CD, Jenuwein T. 2016. The molecular hallmarks of epigenetic control. Nat. Rev. Genet. 17:487–500 - PubMed
-
- Aoyagi S, Wade PA, Hayes JJ. 2003. Nucleosome sliding induced by the xMi-2 complex does not occur exclusively via a simple twist-diffusion mechanism. J. Biol. Chem. 278:30562–8 - PubMed
Publication types
MeSH terms
Substances
Grants and funding
LinkOut - more resources
Full Text Sources
Other Literature Sources
Research Materials
Miscellaneous