ProAlanase is an Effective Alternative to Trypsin for Proteomics Applications and Disulfide Bond Mapping
- PMID: 33020190
- PMCID: PMC7710147
- DOI: 10.1074/mcp.TIR120.002129
ProAlanase is an Effective Alternative to Trypsin for Proteomics Applications and Disulfide Bond Mapping
Abstract
Trypsin is the protease of choice in bottom-up proteomics. However, its application can be limited by the amino acid composition of target proteins and the pH of the digestion solution. In this study we characterize ProAlanase, a protease from the fungus Aspergillus niger that cleaves primarily on the C-terminal side of proline and alanine residues. ProAlanase achieves high proteolytic activity and specificity when digestion is carried out at acidic pH (1.5) for relatively short (2 h) time periods. To elucidate the potential of ProAlanase in proteomics applications, we conducted a series of investigations comprising comparative multi-enzymatic profiling of a human cell line proteome, histone PTM analysis, ancient bone protein identification, phosphosite mapping and de novo sequencing of a proline-rich protein and disulfide bond mapping in mAb. The results demonstrate that ProAlanase is highly suitable for proteomics analysis of the arginine- and lysine-rich histones, enabling high sequence coverage of multiple histone family members. It also facilitates an efficient digestion of bone collagen thanks to the cleavage at the C terminus of hydroxyproline which is highly prevalent in collagen. This allows to identify complementary proteins in ProAlanase- and trypsin-digested ancient bone samples, as well as to increase sequence coverage of noncollagenous proteins. Moreover, digestion with ProAlanase improves protein sequence coverage and phosphosite localization for the proline-rich protein Notch3 intracellular domain (N3ICD). Furthermore, we achieve a nearly complete coverage of N3ICD protein by de novo sequencing using the combination of ProAlanase and tryptic peptides. Finally, we demonstrate that ProAlanase is efficient in disulfide bond mapping, showing high coverage of disulfide-containing regions in a nonreduced mAb.
Keywords: De novo sequencing; Disulfides*; Histones*; PTMs; Phosphoproteins*; ProAlanase; Proline-rich Proteins; Proteases*; Proteolysis*.
© 2020 Samodova et al.
Conflict of interest statement
Conflict of interest—The authors have declared a conflict of interest. Christopher Hosfield and Michael Rosenblatt are employees of Promega Corporation. Promega plans to sell sequencing grade ProAlanase for proteomics. Christian N. Cramer is an employee of Novo Nordisk a/s.
Figures
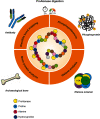
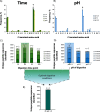
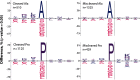
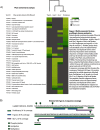
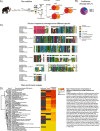
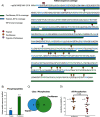
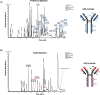
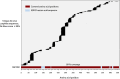
Similar articles
-
Targeting proline in (phospho)proteomics.FEBS J. 2020 Jul;287(14):2979-2997. doi: 10.1111/febs.15190. Epub 2020 Jan 13. FEBS J. 2020. PMID: 31863553 Free PMC article.
-
The utility of proteases in proteomics, from sequence profiling to structure and function analysis.Proteomics. 2023 Mar;23(6):e2200132. doi: 10.1002/pmic.202200132. Epub 2022 Nov 20. Proteomics. 2023. PMID: 36382392 Review.
-
Proteomics beyond trypsin.FEBS J. 2015 Jul;282(14):2612-26. doi: 10.1111/febs.13287. Epub 2015 Apr 14. FEBS J. 2015. PMID: 25823410 Review.
-
Expanding proteome coverage with orthogonal-specificity α-lytic proteases.Mol Cell Proteomics. 2014 Mar;13(3):823-35. doi: 10.1074/mcp.M113.034710. Epub 2014 Jan 14. Mol Cell Proteomics. 2014. PMID: 24425750 Free PMC article.
-
Dual matrix-based immobilized trypsin for complementary proteolytic digestion and fast proteomics analysis with higher protein sequence coverage.Anal Chem. 2014 Feb 4;86(3):1452-8. doi: 10.1021/ac402696b. Epub 2014 Jan 21. Anal Chem. 2014. PMID: 24447065
Cited by
-
Sequential trypsin and ProAlanase digestions unearth immunological protein biomarkers shrouded by skeletal collagen.iScience. 2024 Apr 3;27(5):109663. doi: 10.1016/j.isci.2024.109663. eCollection 2024 May 17. iScience. 2024. PMID: 38655200 Free PMC article.
-
Proteomics of the heart.Physiol Rev. 2024 Jul 1;104(3):931-982. doi: 10.1152/physrev.00026.2023. Epub 2024 Feb 1. Physiol Rev. 2024. PMID: 38300522 Free PMC article. Review.
-
Structural and time-resolved mechanistic investigations of protein hydrolysis by the acidic proline-specific endoprotease from Aspergillus niger.Protein Sci. 2024 Jan;33(1):e4856. doi: 10.1002/pro.4856. Protein Sci. 2024. PMID: 38059672 Free PMC article.
-
A Strategy to Characterize the Global Landscape of Histone Post-Translational Modifications Within Tissues of Nonmodel Organisms.J Proteome Res. 2024 Aug 2;23(8):2780-2794. doi: 10.1021/acs.jproteome.3c00246. Epub 2023 Aug 25. J Proteome Res. 2024. PMID: 37624673 Free PMC article.
-
Cross-linking mass spectrometry discovers, evaluates, and corroborates structures and protein-protein interactions in the human cell.Proc Natl Acad Sci U S A. 2023 Apr 25;120(17):e2219418120. doi: 10.1073/pnas.2219418120. Epub 2023 Apr 18. Proc Natl Acad Sci U S A. 2023. PMID: 37071682 Free PMC article.
References
-
- Olsen J. V., Ong S.-E., and Mann M. (2004) Trypsin cleaves exclusively C-terminal to arginine and lysine residues. Mol. Cell. Proteomics 3, 608–614 - PubMed
Publication types
MeSH terms
Substances
LinkOut - more resources
Full Text Sources
Miscellaneous