Opportunities of connectomic neuromodulation
- PMID: 32702488
- PMCID: PMC7847552
- DOI: 10.1016/j.neuroimage.2020.117180
Opportunities of connectomic neuromodulation
Abstract
The process of altering neural activity - neuromodulation - has long been used to treat patients with brain disorders and answer scientific questions. Deep brain stimulation in particular has provided clinical benefit to over 150,000 patients. However, our understanding of how neuromodulation impacts the brain is evolving. Instead of focusing on the local impact at the stimulation site itself, we are considering the remote impact on brain regions connected to the stimulation site. Brain connectivity information derived from advanced magnetic resonance imaging data can be used to identify these connections and better understand clinical and behavioral effects of neuromodulation. In this article, we review studies combining neuromodulation and brain connectomics, highlighting opportunities where this approach may prove particularly valuable. We focus on deep brain stimulation, but show that the same principles can be applied to other forms of neuromodulation, such as transcranial magnetic stimulation and MRI-guided focused ultrasound. We outline future perspectives and provide testable hypotheses for future work.
Keywords: Brain networks; Brain stimulation; Connectomics; Deep brain stimulation; Diffusion MRI; Functional MRI; Network fingerprinting; Neuromodulation; Tractography; dMRI; fMRI.
Copyright © 2020 The Author(s). Published by Elsevier Inc. All rights reserved.
Figures
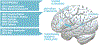
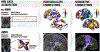
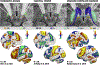
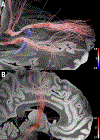
Similar articles
-
Connectomic Deep Brain Stimulation for Obsessive-Compulsive Disorder.Biol Psychiatry. 2021 Nov 15;90(10):678-688. doi: 10.1016/j.biopsych.2021.07.010. Epub 2021 Jul 19. Biol Psychiatry. 2021. PMID: 34482949 Review.
-
A systematic review of preclinical and clinical transcranial ultrasound neuromodulation and opportunities for functional connectomics.Brain Stimul. 2024 Jul-Aug;17(4):734-751. doi: 10.1016/j.brs.2024.06.005. Epub 2024 Jun 14. Brain Stimul. 2024. PMID: 38880207
-
Neuromodulation for brain disorders: challenges and opportunities.IEEE Trans Biomed Eng. 2013 Mar;60(3):610-24. doi: 10.1109/TBME.2013.2244890. Epub 2013 Feb 1. IEEE Trans Biomed Eng. 2013. PMID: 23380851 Free PMC article. Review.
-
Diffusion and functional MRI in surgical neuromodulation.Neurotherapeutics. 2024 Apr;21(3):e00364. doi: 10.1016/j.neurot.2024.e00364. Epub 2024 Apr 25. Neurotherapeutics. 2024. PMID: 38669936 Free PMC article. Review.
-
Neuromodulation for Pain: A Comprehensive Survey and Systematic Review of Clinical Trials and Connectomic Analysis of Brain Targets.Stereotact Funct Neurosurg. 2022;100(1):14-25. doi: 10.1159/000517873. Epub 2021 Aug 11. Stereotact Funct Neurosurg. 2022. PMID: 34380132 Review.
Cited by
-
Comparing structure-function relationships in brain networks using EEG and fNIRS.Sci Rep. 2024 Nov 22;14(1):28976. doi: 10.1038/s41598-024-79817-x. Sci Rep. 2024. PMID: 39578593 Free PMC article.
-
Effects of anodal transcranial direct current stimulation on postural stability in subacute stroke: A randomized control trial.Physiol Rep. 2024 Oct;12(19):e70067. doi: 10.14814/phy2.70067. Physiol Rep. 2024. PMID: 39349984 Free PMC article. Clinical Trial.
-
Brain Networks for Cortical Atrophy and Responsive Neurostimulation in Temporal Lobe Epilepsy.JAMA Neurol. 2024 Sep 30;81(11):1199-209. doi: 10.1001/jamaneurol.2024.2952. Online ahead of print. JAMA Neurol. 2024. PMID: 39348148
-
The Neurostimulationist will see you now: prescribing direct electrical stimulation therapies for the human brain in epilepsy and beyond.Front Hum Neurosci. 2024 Sep 4;18:1439541. doi: 10.3389/fnhum.2024.1439541. eCollection 2024. Front Hum Neurosci. 2024. PMID: 39296917 Free PMC article. Review.
-
Multimodal neuroimaging to characterize symptom-specific networks in movement disorders.NPJ Parkinsons Dis. 2024 Aug 14;10(1):154. doi: 10.1038/s41531-024-00774-3. NPJ Parkinsons Dis. 2024. PMID: 39143114 Free PMC article. Review.
References
-
- Ackermans L, Duits A, van der Linden C, Tijssen M, Schruers K, Temel Y, Kleijer M, Nederveen P, Bruggeman R, Tromp S, van Kranen-Mastenbroek V, Kingma H, Cath D, Visser-Vandewalle V, 2011. Double-blind clinical trial of thalamic stimulation in patients with Tourette syndrome. Brain 134, 832–844. doi:10.1093/brain/awq380. - DOI - PubMed
-
- Akram H, Dayal V, Mahlknecht P, Georgiev D, Hyam J, Foltynie T, Limousin P, De Vita E, Jahanshahi M, Ashburner J, Behrens T, Hariz M, Zrinzo L, 2018. Connectivity derived thalamic segmentation in deep brain stimulation for tremor. Neuroimage Clin. 18, 130–142. doi:10.1016/j.nicl.2018.01.008. - DOI - PMC - PubMed
-
- Akram H, Sotiropoulos SN, Jbabdi S, Georgiev D, Mahlknecht P, Hyam J, Foltynie T, Limousin P, De Vita E, Jahanshahi M, Hariz M, Ashburner J, Behrens T, Zrinzo L, 2017. Subthalamic deep brain stimulation sweet spots and hyperdirect cortical connectivity in Parkinson’s disease. NeuroImage 158, 332–345. doi:10.1016/j.neuroimage.2017.07.012. - DOI - PMC - PubMed
Publication types
MeSH terms
Grants and funding
LinkOut - more resources
Full Text Sources
Other Literature Sources
Medical