Sodium Channel SCN3A (NaV1.3) Regulation of Human Cerebral Cortical Folding and Oral Motor Development
- PMID: 30146301
- PMCID: PMC6226006
- DOI: 10.1016/j.neuron.2018.07.052
Sodium Channel SCN3A (NaV1.3) Regulation of Human Cerebral Cortical Folding and Oral Motor Development
Abstract
Channelopathies are disorders caused by abnormal ion channel function in differentiated excitable tissues. We discovered a unique neurodevelopmental channelopathy resulting from pathogenic variants in SCN3A, a gene encoding the voltage-gated sodium channel NaV1.3. Pathogenic NaV1.3 channels showed altered biophysical properties including increased persistent current. Remarkably, affected individuals showed disrupted folding (polymicrogyria) of the perisylvian cortex of the brain but did not typically exhibit epilepsy; they presented with prominent speech and oral motor dysfunction, implicating SCN3A in prenatal development of human cortical language areas. The development of this disorder parallels SCN3A expression, which we observed to be highest early in fetal cortical development in progenitor cells of the outer subventricular zone and cortical plate neurons and decreased postnatally, when SCN1A (NaV1.1) expression increased. Disrupted cerebral cortical folding and neuronal migration were recapitulated in ferrets expressing the mutant channel, underscoring the unexpected role of SCN3A in progenitor cells and migrating neurons.
Keywords: Cortical Development; Na(V)1.1; Na(V)1.3; Oromotor; Outer Radial Glia; Polymicrogyria; SCN1A; SCN3A; Speech; Voltage-Gated Sodium Channel (VGSC).
Copyright © 2018 Elsevier Inc. All rights reserved.
Conflict of interest statement
DECLARATION OF INTERESTS
The authors declare no competing interests.
Figures
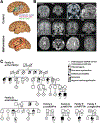
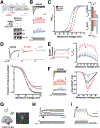
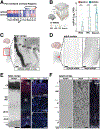
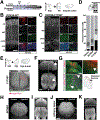
Comment in
-
Gyrification Needs Correct Sodium Flux!Neuron. 2018 Sep 5;99(5):867-868. doi: 10.1016/j.neuron.2018.08.005. Neuron. 2018. PMID: 30189204
-
An Electric Take on Neural Fate and Cortical Development.Dev Cell. 2019 Jan 7;48(1):1-2. doi: 10.1016/j.devcel.2018.12.014. Dev Cell. 2019. PMID: 30620896
Similar articles
-
SCN3A-Related Neurodevelopmental Disorder: A Spectrum of Epilepsy and Brain Malformation.Ann Neurol. 2020 Aug;88(2):348-362. doi: 10.1002/ana.25809. Epub 2020 Jul 9. Ann Neurol. 2020. PMID: 32515017 Free PMC article.
-
Neurodevelopmental disorder associated with de novo SCN3A pathogenic variants: two new cases and review of the literature.Brain Dev. 2020 Feb;42(2):211-216. doi: 10.1016/j.braindev.2019.09.004. Epub 2019 Oct 31. Brain Dev. 2020. PMID: 31677917 Review.
-
Mutations in SCN3A cause early infantile epileptic encephalopathy.Ann Neurol. 2018 Apr;83(4):703-717. doi: 10.1002/ana.25188. Epub 2018 Mar 30. Ann Neurol. 2018. PMID: 29466837 Free PMC article.
-
Targeted blockade of aberrant sodium current in a stem cell-derived neuron model of SCN3A encephalopathy.Brain. 2024 Apr 4;147(4):1247-1263. doi: 10.1093/brain/awad376. Brain. 2024. PMID: 37935051 Free PMC article.
-
Structure and Function of Sodium Channel Nav1.3 in Neurological Disorders.Cell Mol Neurobiol. 2023 Mar;43(2):575-584. doi: 10.1007/s10571-022-01211-w. Epub 2022 Mar 24. Cell Mol Neurobiol. 2023. PMID: 35332400 Review.
Cited by
-
Gene therapy for targeting a prenatally enriched potassium channel associated with severe childhood epilepsy and premature death.bioRxiv [Preprint]. 2024 Oct 24:2024.10.24.620125. doi: 10.1101/2024.10.24.620125. bioRxiv. 2024. PMID: 39484453 Free PMC article. Preprint.
-
Developmental and epileptic encephalopathies.Nat Rev Dis Primers. 2024 Sep 5;10(1):61. doi: 10.1038/s41572-024-00546-6. Nat Rev Dis Primers. 2024. PMID: 39237642 Review.
-
Shining a Light on Venom-Peptide Receptors: Venom Peptides as Targeted Agents for In Vivo Molecular Imaging.Toxins (Basel). 2024 Jul 4;16(7):307. doi: 10.3390/toxins16070307. Toxins (Basel). 2024. PMID: 39057947 Free PMC article. Review.
-
Heterogeneous genetic patterns in bilateral perisylvian polymicrogyria: insights from a Finnish family cohort.Brain Commun. 2024 Apr 18;6(3):fcae142. doi: 10.1093/braincomms/fcae142. eCollection 2024. Brain Commun. 2024. PMID: 38712318 Free PMC article. Review.
-
Channelopathies in epilepsy: an overview of clinical presentations, pathogenic mechanisms, and therapeutic insights.J Neurol. 2024 Jun;271(6):3063-3094. doi: 10.1007/s00415-024-12352-x. Epub 2024 Apr 12. J Neurol. 2024. PMID: 38607431 Review.
References
-
- Adam MP, Ardinger HH, Pagon RA, Wallace SE, Bean LJ, Mefford HC, Stephens K, Amemiya A, Ledbetter N, Morgan A, Fisher SE, Scheffer I, Hildebrand M, (1993). FOXP2-Related Speech and Language Disorders.
-
- Ben-Shalom R, Keeshen CM, Berrios KN, An JY, Sanders SJ, Bender KJ, (2017). Opposing Effects on NaV1.2 Function Underlie Differences Between SCN2A Variants Observed in Individuals With Autism Spectrum Disorder or Infantile Seizures. Biol. Psychiatry 0. doi:10.1016/j.biopsych.2017.01.009 - DOI - PMC - PubMed
Publication types
MeSH terms
Substances
Grants and funding
LinkOut - more resources
Full Text Sources
Other Literature Sources
Molecular Biology Databases
Miscellaneous