Rapid conversion of an oceanic spreading center to a subduction zone inferred from high-precision geochronology
- PMID: 27821756
- PMCID: PMC5127376
- DOI: 10.1073/pnas.1609999113
Rapid conversion of an oceanic spreading center to a subduction zone inferred from high-precision geochronology
Abstract
Where and how subduction zones initiate is a fundamental tectonic problem, yet there are few well-constrained geologic tests that address the tectonic settings and dynamics of the process. Numerical modeling has shown that oceanic spreading centers are some of the weakest parts of the plate tectonic system [Gurnis M, Hall C, Lavier L (2004) Geochem Geophys Geosys 5:Q07001], but previous studies have not favored them for subduction initiation because of the positive buoyancy of young lithosphere. Instead, other weak zones, such as fracture zones, have been invoked. Because these models differ in terms of the ages of crust that are juxtaposed at the site of subduction initiation, they can be tested by dating the protoliths of metamorphosed oceanic crust that is formed by underthrusting at the beginning of subduction and comparing that age with the age of the overlying lithosphere and the timing of subduction initiation itself. In the western Philippines, we find that oceanic crust was less than ∼1 My old when it was underthrust and metamorphosed at the onset of subduction in Palawan, Philippines, implying forced subduction initiation at a spreading center. This result shows that young and positively buoyant, but weak, lithosphere was the preferred site for subduction nucleation despite the proximity of other potential weak zones with older, denser lithosphere and that plate motion rapidly changed from divergence to convergence.
Keywords: Philippines; geochronology; ophiolite; subduction initiation; tectonics.
Conflict of interest statement
The authors declare no conflict of interest.
Figures
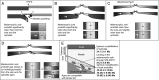
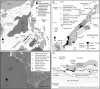
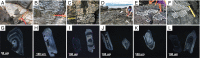
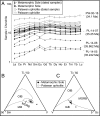
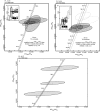

Similar articles
-
Plate tectonics on the Earth triggered by plume-induced subduction initiation.Nature. 2015 Nov 12;527(7577):221-5. doi: 10.1038/nature15752. Nature. 2015. PMID: 26560300
-
Spreading continents kick-started plate tectonics.Nature. 2014 Sep 18;513(7518):405-8. doi: 10.1038/nature13728. Nature. 2014. PMID: 25230662
-
Subduction-driven recycling of continental margin lithosphere.Nature. 2014 Nov 13;515(7526):253-6. doi: 10.1038/nature13878. Nature. 2014. PMID: 25391963
-
The inception of plate tectonics: a record of failure.Philos Trans A Math Phys Eng Sci. 2018 Oct 1;376(2132):20170414. doi: 10.1098/rsta.2017.0414. Philos Trans A Math Phys Eng Sci. 2018. PMID: 30275162 Free PMC article. Review.
-
Indian plate paleogeography, subduction and horizontal underthrusting below Tibet: paradoxes, controversies and opportunities.Natl Sci Rev. 2022 Apr 21;9(8):nwac074. doi: 10.1093/nsr/nwac074. eCollection 2022 Aug. Natl Sci Rev. 2022. PMID: 35992242 Free PMC article. Review.
Cited by
-
Boron isotopes in boninites document rapid changes in slab inputs during subduction initiation.Nat Commun. 2022 Feb 22;13(1):993. doi: 10.1038/s41467-022-28637-6. Nat Commun. 2022. PMID: 35194052 Free PMC article.
-
Differentiating induced versus spontaneous subduction initiation using thermomechanical models and metamorphic soles.Nat Commun. 2021 Jul 30;12(1):4632. doi: 10.1038/s41467-021-24896-x. Nat Commun. 2021. PMID: 34330929 Free PMC article.
-
Intraoceanic subduction spanned the Pacific in the Late Cretaceous-Paleocene.Sci Adv. 2017 Nov 8;3(11):eaao2303. doi: 10.1126/sciadv.aao2303. eCollection 2017 Nov. Sci Adv. 2017. PMID: 29134200 Free PMC article.
References
-
- Forsyth D, Uyeda S. On the relative importance of the driving forces of plate motion. Geophys J R Astron Soc. 1975;43(1):163–200.
-
- Gurnis M, Hall C, Lavier L. Evolving force balance during incipient subduction. Geochem Geophys Geosyst. 2004;5(7):Q07001.
-
- McKenzie DP. 1977. The initiation of trenches: A finite amplitude instability. Island Arcs, Deep Sea Trenches and Back-Arc Basins, Maurice Ewing Series, eds Talwani M, Pitman WC IIII (American Geophysical Union, Washington, DC), Vol 1, pp 57–61.
-
- Mueller S, Phillips RJ. On the initiation of subduction. J Geophys Res. 1991;96(B1):651–665.
-
- Toth J, Gurnis M. Dynamics of subduction initiation at preexisting fault zones. J Geophys Res. 1998;103(B8):18053–18067.
Publication types
LinkOut - more resources
Full Text Sources
Other Literature Sources