A de novo compound targeting α-synuclein improves deficits in models of Parkinson's disease
- PMID: 27679481
- PMCID: PMC5840882
- DOI: 10.1093/brain/aww238
A de novo compound targeting α-synuclein improves deficits in models of Parkinson's disease
Erratum in
-
Correction to: A de novo compound targeting α-synuclein improves deficits in models of Parkinson's disease.Brain. 2023 Oct 3;146(10):e92-e93. doi: 10.1093/brain/awad207. Brain. 2023. PMID: 37350503 No abstract available.
Abstract
Abnormal accumulation and propagation of the neuronal protein α-synuclein has been hypothesized to underlie the pathogenesis of Parkinson's disease, dementia with Lewy bodies and multiple system atrophy. Here we report a de novo-developed compound (NPT100-18A) that reduces α-synuclein toxicity through a novel mechanism that involves displacing α-synuclein from the membrane. This compound interacts with a domain in the C-terminus of α-synuclein. The E83R mutation reduces the compound interaction with the 80-90 amino acid region of α-synuclein and prevents the effects of NPT100-18A. In vitro studies showed that NPT100-18A reduced the formation of wild-type α-synuclein oligomers in membranes, reduced the neuronal accumulation of α-synuclein, and decreased markers of cell toxicity. In vivo studies were conducted in three different α-synuclein transgenic rodent models. Treatment with NPT100-18A ameliorated motor deficits in mThy1 wild-type α-synuclein transgenic mice in a dose-dependent manner at two independent institutions. Neuropathological examination showed that NPT100-18A decreased the accumulation of proteinase K-resistant α-synuclein aggregates in the CNS and was accompanied by the normalization of neuronal and inflammatory markers. These results were confirmed in a mutant line of α-synuclein transgenic mice that is prone to generate oligomers. In vivo imaging studies of α-synuclein-GFP transgenic mice using two-photon microscopy showed that NPT100-18A reduced the cortical synaptic accumulation of α-synuclein within 1 h post-administration. Taken together, these studies support the notion that altering the interaction of α-synuclein with the membrane might be a feasible therapeutic approach for developing new disease-modifying treatments of Parkinson's disease and other synucleinopathies.
Keywords: Parkinson’s disease; alpha-synuclein; cellular mechanisms; experimental models; synucleinopathy.
© The Author (2016). Published by Oxford University Press on behalf of the Guarantors of Brain. All rights reserved. For Permissions, please email: journals.permissions@oup.com.
Figures
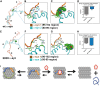
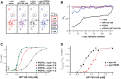
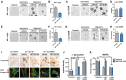
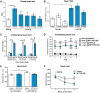
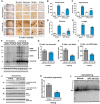
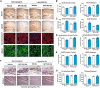
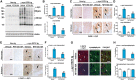
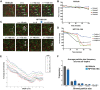
Comment in
-
A novel therapeutic approach for synucleinopathies.Mov Disord. 2016 Dec;31(12):1797. doi: 10.1002/mds.26871. Epub 2016 Nov 21. Mov Disord. 2016. PMID: 27869325 No abstract available.
Similar articles
-
Accumulation of oligomer-prone α-synuclein exacerbates synaptic and neuronal degeneration in vivo.Brain. 2014 May;137(Pt 5):1496-513. doi: 10.1093/brain/awu057. Epub 2014 Mar 24. Brain. 2014. PMID: 24662516 Free PMC article.
-
Longitudinal live imaging of retinal α-synuclein::GFP deposits in a transgenic mouse model of Parkinson's Disease/Dementia with Lewy Bodies.Sci Rep. 2016 Jul 8;6:29523. doi: 10.1038/srep29523. Sci Rep. 2016. PMID: 27389831 Free PMC article.
-
Development of an α-synuclein knockdown peptide and evaluation of its efficacy in Parkinson's disease models.Commun Biol. 2021 Feb 19;4(1):232. doi: 10.1038/s42003-021-01746-6. Commun Biol. 2021. PMID: 33608634 Free PMC article.
-
Immunotherapy targeting α-synuclein, with relevance for future treatment of Parkinson's disease and other Lewy body disorders.Immunotherapy. 2014;6(2):141-53. doi: 10.2217/imt.13.162. Immunotherapy. 2014. PMID: 24491088 Review.
-
Activating Autophagy as a Therapeutic Strategy for Parkinson's Disease.CNS Drugs. 2018 Jan;32(1):1-11. doi: 10.1007/s40263-018-0497-5. CNS Drugs. 2018. PMID: 29492779 Review.
Cited by
-
Parkinson disease therapy: current strategies and future research priorities.Nat Rev Neurol. 2024 Nov 4. doi: 10.1038/s41582-024-01034-x. Online ahead of print. Nat Rev Neurol. 2024. PMID: 39496848 Review.
-
α-synuclein overexpression and the microbiome shape the gut and brain metabolome in mice.NPJ Parkinsons Dis. 2024 Oct 30;10(1):208. doi: 10.1038/s41531-024-00816-w. NPJ Parkinsons Dis. 2024. PMID: 39477976 Free PMC article.
-
Trends on Novel Targets and Nanotechnology-Based Drug Delivery System in the Treatment of Parkinson's disease: Recent Advancement in Drug Development.Curr Drug Targets. 2024;25(15):987-1011. doi: 10.2174/0113894501312703240826070530. Curr Drug Targets. 2024. PMID: 39313872 Review.
-
α-Synuclein Overexpression and the Microbiome Shape the Gut and Brain Metabolome in Mice.bioRxiv [Preprint]. 2024 Jun 10:2024.06.07.597975. doi: 10.1101/2024.06.07.597975. bioRxiv. 2024. Update in: NPJ Parkinsons Dis. 2024 Oct 30;10(1):208. doi: 10.1038/s41531-024-00816-w. PMID: 38915679 Free PMC article. Updated. Preprint.
-
Multiple system atrophy: an update and emerging directions of biomarkers and clinical trials.J Neurol. 2024 May;271(5):2324-2344. doi: 10.1007/s00415-024-12269-5. Epub 2024 Mar 14. J Neurol. 2024. PMID: 38483626 Free PMC article. Review.
References
-
- Alvarez-Castelao B, Goethals M, Vandekerckhove J, Castano JG. Mechanism of cleavage of alpha-synuclein by the 20S proteasome and modulation of its degradation by the RedOx state of the N-terminal methionines. Biochim Biophys Acta 2014; 1843: 352–65. - PubMed
-
- Beyer K. Mechanistic aspects of Parkinson's disease: alpha-synuclein and the biomembrane. Cell Biochem Biophys 2007; 47: 285–99. - PubMed
Publication types
MeSH terms
Substances
Grants and funding
LinkOut - more resources
Full Text Sources
Other Literature Sources
Medical