The rainbow trout genome provides novel insights into evolution after whole-genome duplication in vertebrates
- PMID: 24755649
- PMCID: PMC4071752
- DOI: 10.1038/ncomms4657
The rainbow trout genome provides novel insights into evolution after whole-genome duplication in vertebrates
Abstract
Vertebrate evolution has been shaped by several rounds of whole-genome duplications (WGDs) that are often suggested to be associated with adaptive radiations and evolutionary innovations. Due to an additional round of WGD, the rainbow trout genome offers a unique opportunity to investigate the early evolutionary fate of a duplicated vertebrate genome. Here we show that after 100 million years of evolution the two ancestral subgenomes have remained extremely collinear, despite the loss of half of the duplicated protein-coding genes, mostly through pseudogenization. In striking contrast is the fate of miRNA genes that have almost all been retained as duplicated copies. The slow and stepwise rediploidization process characterized here challenges the current hypothesis that WGD is followed by massive and rapid genomic reorganizations and gene deletions.
Figures
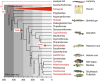
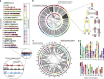
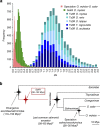
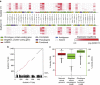
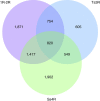
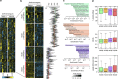
Similar articles
-
Distribution of ancestral proto-Actinopterygian chromosome arms within the genomes of 4R-derivative salmonid fishes (Rainbow trout and Atlantic salmon).BMC Genomics. 2008 Nov 25;9:557. doi: 10.1186/1471-2164-9-557. BMC Genomics. 2008. PMID: 19032764 Free PMC article.
-
Pck-ing up steam: Widening the salmonid gluconeogenic gene duplication trail.Gene. 2019 May 25;698:129-140. doi: 10.1016/j.gene.2019.02.079. Epub 2019 Mar 5. Gene. 2019. PMID: 30849535
-
Evolutionary history of DNA methylation related genes in chordates: new insights from multiple whole genome duplications.Sci Rep. 2020 Jan 22;10(1):970. doi: 10.1038/s41598-020-57753-w. Sci Rep. 2020. PMID: 31969623 Free PMC article.
-
Impact of gene gains, losses and duplication modes on the origin and diversification of vertebrates.Semin Cell Dev Biol. 2013 Feb;24(2):83-94. doi: 10.1016/j.semcdb.2012.12.008. Epub 2013 Jan 3. Semin Cell Dev Biol. 2013. PMID: 23291262 Review.
-
Functional Divergence between Subgenomes and Gene Pairs after Whole Genome Duplications.Mol Plant. 2018 Mar 5;11(3):388-397. doi: 10.1016/j.molp.2017.12.010. Epub 2017 Dec 22. Mol Plant. 2018. PMID: 29275166 Review.
Cited by
-
An improved transcriptome annotation reveals asymmetric expression and distinct regulation patterns in allotetraploid common carp.Commun Biol. 2024 Nov 20;7(1):1542. doi: 10.1038/s42003-024-07177-3. Commun Biol. 2024. PMID: 39567764 Free PMC article.
-
Exploring Fish Parvalbumins through Allergen Names and Gene Identities.Genes (Basel). 2024 Oct 18;15(10):1337. doi: 10.3390/genes15101337. Genes (Basel). 2024. PMID: 39457461 Free PMC article. Review.
-
Genome-wide identification and expression profiling of the Wnt gene family in three abalone species.Genes Genomics. 2024 Oct 14. doi: 10.1007/s13258-024-01579-7. Online ahead of print. Genes Genomics. 2024. PMID: 39397130 Review.
-
Evolution of Key Oxygen-Sensing Genes Is Associated with Hypoxia Tolerance in Fishes.Genome Biol Evol. 2024 Sep 3;16(9):evae183. doi: 10.1093/gbe/evae183. Genome Biol Evol. 2024. PMID: 39165136 Free PMC article.
-
Decoding the fish genome opens a new era in important trait research and molecular breeding in China.Sci China Life Sci. 2024 Oct;67(10):2064-2083. doi: 10.1007/s11427-023-2670-5. Epub 2024 Aug 12. Sci China Life Sci. 2024. PMID: 39145867 Review.
References
-
- Ohno S. Evolution by Gene Duplication Allen and Unwin (1970).
-
- Jaillon O. et al. Genome duplication in the teleost fish Tetraodon nigroviridis reveals the early vertebrate proto-karyotype. Nature 431, 946–957 (2004). - PubMed
-
- Amores A. et al. Zebrafish hox clusters and vertebrate genome evolution. Science 282, 1711–1714 (1998). - PubMed
Publication types
MeSH terms
Associated data
LinkOut - more resources
Full Text Sources
Other Literature Sources
Molecular Biology Databases