Quorum sensing: how bacteria can coordinate activity and synchronize their response to external signals?
- PMID: 22825856
- PMCID: PMC3526984
- DOI: 10.1002/pro.2132
Quorum sensing: how bacteria can coordinate activity and synchronize their response to external signals?
Abstract
Quorum sensing is used by a large variety of bacteria to regulate gene expression in a cell-density-dependent manner. Bacteria can synchronize population behavior using small molecules called autoinducers that are produced by cognate synthases and recognized by specific receptors. Quorum sensing plays critical roles in regulating diverse cellular functions in bacteria, including bioluminescence, virulence gene expression, biofilm formation, and antibiotic resistance. The best-studied autoinducers are acyl homoserine lactone (AHL) molecules, which are the primary quorum sensing signals used by Gram-negative bacteria. In this review we focus on the AHL-dependent quorum sensing system and highlight recent progress on structural and mechanistic studies of AHL synthases and the corresponding receptors. Crystal structures of LuxI-type AHL synthases provide insights into acyl-substrate specificity, but the current knowledge is still greatly limited. Structural studies of AHL receptors have facilitated a more thorough understanding of signal perception and established the molecular framework for the development of quorum sensing inhibitors.
Copyright © 2012 The Protein Society.
Figures
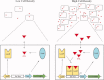
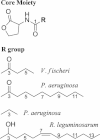
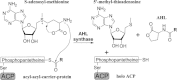
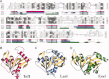
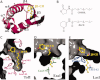
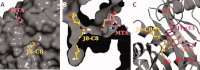
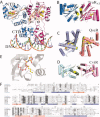
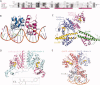
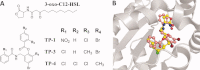
Similar articles
-
N-Acyl Homoserine Lactone Analog Modulators of the Pseudomonas aeruginosa Rhll Quorum Sensing Signal Synthase.ACS Chem Biol. 2019 Oct 18;14(10):2305-2314. doi: 10.1021/acschembio.9b00671. Epub 2019 Oct 9. ACS Chem Biol. 2019. PMID: 31545595 Free PMC article.
-
Molecular Mechanisms and Applications of N-Acyl Homoserine Lactone-Mediated Quorum Sensing in Bacteria.Molecules. 2022 Nov 4;27(21):7584. doi: 10.3390/molecules27217584. Molecules. 2022. PMID: 36364411 Free PMC article. Review.
-
An evolving perspective on the Pseudomonas aeruginosa orphan quorum sensing regulator QscR.Front Cell Infect Microbiol. 2014 Oct 28;4:152. doi: 10.3389/fcimb.2014.00152. eCollection 2014. Front Cell Infect Microbiol. 2014. PMID: 25389523 Free PMC article. Review.
-
Signal Integration in Quorum Sensing Enables Cross-Species Induction of Virulence in Pectobacterium wasabiae.mBio. 2017 May 23;8(3):e00398-17. doi: 10.1128/mBio.00398-17. mBio. 2017. PMID: 28536283 Free PMC article.
-
Enzymatic Assays to Investigate Acyl-Homoserine Lactone Autoinducer Synthases.Methods Mol Biol. 2018;1673:161-176. doi: 10.1007/978-1-4939-7309-5_13. Methods Mol Biol. 2018. PMID: 29130172 Free PMC article.
Cited by
-
Programmable Bacteria with Dynamic Virulence Modulation System for Precision Antitumor Immunity.Adv Sci (Weinh). 2024 Sep;11(36):e2404069. doi: 10.1002/advs.202404069. Epub 2024 Jul 26. Adv Sci (Weinh). 2024. PMID: 39058336 Free PMC article.
-
Beyond antibiotics: CRISPR/Cas9 triumph over biofilm-associated antibiotic resistance infections.Front Cell Infect Microbiol. 2024 Jul 5;14:1408569. doi: 10.3389/fcimb.2024.1408569. eCollection 2024. Front Cell Infect Microbiol. 2024. PMID: 39035353 Free PMC article. Review.
-
Roles and Regulation of Quorum Sensing of Acidophiles in Bioleaching: A Review.Microorganisms. 2024 Feb 20;12(3):422. doi: 10.3390/microorganisms12030422. Microorganisms. 2024. PMID: 38543473 Free PMC article. Review.
-
Effect of Matricaria aurea Essential Oils on Biofilm Development, Virulence Factors and Quorum Sensing-Dependent Genes of Pseudomonas aeruginosa.Pharmaceuticals (Basel). 2024 Mar 18;17(3):386. doi: 10.3390/ph17030386. Pharmaceuticals (Basel). 2024. PMID: 38543172 Free PMC article.
-
Shifting from Ammonium to Phosphonium Salts: A Promising Strategy to Develop Next-Generation Weapons against Biofilms.Pharmaceutics. 2024 Jan 5;16(1):80. doi: 10.3390/pharmaceutics16010080. Pharmaceutics. 2024. PMID: 38258091 Free PMC article. Review.
References
Publication types
MeSH terms
Substances
LinkOut - more resources
Full Text Sources