Structural determinants of drugs acting on the Nav1.8 channel
- PMID: 19233853
- PMCID: PMC2667739
- DOI: 10.1074/jbc.M807569200
Structural determinants of drugs acting on the Nav1.8 channel
Abstract
The aim of this work is to study the role of pore residues on drug binding in the Na(V)1.8 channel. Alanine mutations were made in the S6 segments, chosen on the basis of their roles in other Na(V) subtypes; whole cell patch clamp recordings were made from mammalian ND7/23 cells. Mutations of some residues caused shifts in voltage dependence of activation and inactivation, and gave faster time course of inactivation, indicating that the residues mutated play important roles in both activation and inactivation in the Na(V)1.8 channel. The resting and inactivated state affinities of tetracaine for the channel were reduced by mutations I381A, F1710A, and Y1717A (for the latter only inactivated state affinity was measured), and by mutation F1710A for the Na(V)1.8-selective compound A-803467, showing the involvement of these residues for each compound, respectively. For both compounds, mutation L1410A caused the unexpected appearance of a complete resting block even at extremely low concentrations. Resting block of native channels by compound A-803467 could be partially removed ("disinhibition") by repetitive stimulation or by a test pulse after recovery from inactivation; the magnitude of the latter effect was increased for all the mutants studied. Tetracaine did not show this effect for native channels, but disinhibition was seen particularly for mutants L1410A and F1710A. The data suggest differing, but partially overlapping, areas of binding of A-803467 and tetracaine. Docking of the ligands into a three-dimensional model of the Na(V)1.8 channel gave interesting insight as to how the ligands may interact with pore residues.
Figures
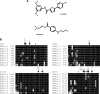
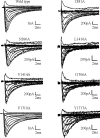
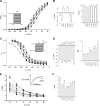
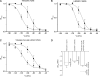
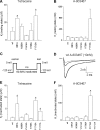
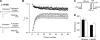
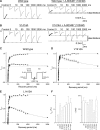
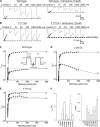
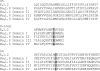
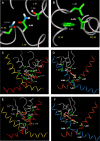
Similar articles
-
Charge at the lidocaine binding site residue Phe-1759 affects permeation in human cardiac voltage-gated sodium channels.J Physiol. 2007 Jun 1;581(Pt 2):741-55. doi: 10.1113/jphysiol.2007.130161. Epub 2007 Mar 15. J Physiol. 2007. PMID: 17363383 Free PMC article.
-
Molecular determinants of voltage-dependent gating and binding of pore-blocking drugs in transmembrane segment IIIS6 of the Na(+) channel alpha subunit.J Biol Chem. 2001 Jan 5;276(1):20-7. doi: 10.1074/jbc.M006992200. J Biol Chem. 2001. PMID: 11024055
-
Residues in Na(+) channel D3-S6 segment modulate both batrachotoxin and local anesthetic affinities.Biophys J. 2000 Sep;79(3):1379-87. doi: 10.1016/S0006-3495(00)76390-9. Biophys J. 2000. PMID: 10969000 Free PMC article.
-
Interactions of local anesthetics with voltage-gated Na+ channels.J Membr Biol. 2004 Sep 1;201(1):1-8. doi: 10.1007/s00232-004-0702-y. J Membr Biol. 2004. PMID: 15635807 Review.
-
Molecular properties of brain sodium channels: an important target for anticonvulsant drugs.Adv Neurol. 1999;79:441-56. Adv Neurol. 1999. PMID: 10514834 Review.
Cited by
-
Use-Dependent Relief of Inhibition of Nav1.8 Channels by A-887826.Mol Pharmacol. 2023 Apr;103(4):221-229. doi: 10.1124/molpharm.122.000593. Epub 2023 Jan 12. Mol Pharmacol. 2023. PMID: 36635052 Free PMC article.
-
Structural basis for high-voltage activation and subtype-specific inhibition of human Nav1.8.Proc Natl Acad Sci U S A. 2022 Jul 26;119(30):e2208211119. doi: 10.1073/pnas.2208211119. Epub 2022 Jul 19. Proc Natl Acad Sci U S A. 2022. PMID: 35858452 Free PMC article.
-
Functional modulation of the human voltage-gated sodium channel NaV1.8 by auxiliary β subunits.Channels (Austin). 2021 Dec;15(1):79-93. doi: 10.1080/19336950.2020.1860399. Channels (Austin). 2021. PMID: 33315536 Free PMC article.
-
Status of peripheral sodium channel blockers for non-addictive pain treatment.Nat Rev Neurol. 2020 Dec;16(12):689-705. doi: 10.1038/s41582-020-00415-2. Epub 2020 Oct 27. Nat Rev Neurol. 2020. PMID: 33110213 Review.
-
Conservation and divergence in NaChBac and NaV1.7 pharmacology reveals novel drug interaction mechanisms.Sci Rep. 2020 Jul 1;10(1):10730. doi: 10.1038/s41598-020-67761-5. Sci Rep. 2020. PMID: 32612253 Free PMC article.
References
-
- Catterall, W. A., Goldin, A. L., and Waxman, S. G. (2005) Pharmacol. Rev. 57 397-409 - PubMed
-
- Hille, B. (2001) Ionic Channels of Excitable Membranes, Sinauer, Sunderland, MA
-
- Catterall, W. A. (2000) Neuron 26 13-25 - PubMed
-
- Yang, N., George, A. L., Jr., and Horn, R. (1996) Neuron 16 113-122 - PubMed
-
- Goldin, A. L., Barchi, R. L., Caldwell, J. H., Hofmann, F., Howe, J. R., Hunter, J. C., Kallen, R. G., Mandel, G., Meisler, M. H., Netter, Y. B., Noda, M., Tamkun, M. M., Waxman, S. G., Wood, J. N., and Catterall, W. A. (2000) Neuron 28 365-368 - PubMed
Publication types
MeSH terms
Substances
Grants and funding
LinkOut - more resources
Full Text Sources