Altered proteome biology of cardiac mitochondria under stress conditions
- PMID: 18484766
- PMCID: PMC3805274
- DOI: 10.1021/pr070371f
Altered proteome biology of cardiac mitochondria under stress conditions
Abstract
Myocardial ischemia-reperfusion induces mitochondrial dysfunction and, depending upon the degree of injury, may lead to cardiac cell death. However, our ability to understand mitochondrial dysfunction has been hindered by an absence of molecular markers defining the various degrees of injury. To address this paucity of knowledge, we sought to characterize the impact of ischemic damage on mitochondrial proteome biology. We hypothesized that ischemic injury induces differential alterations in various mitochondrial subcompartments, that these proteomic changes are specific to the severity of injury, and that they are important to subsequent cellular adaptations to myocardial ischemic injury. Accordingly, an in vitro model of cardiac mitochondria injury in mice was established to examine two stress conditions: reversible injury (induced by mild calcium overload) and irreversible injury (induced by hypotonic stimuli). Both forms of injury had a drastic impact on the proteome biology of cardiac mitochondria. Altered mitochondrial function was concomitant with significant protein loss/shedding from the injured organelles. In the setting of mild calcium overload, mitochondria retained functionality despite the release of numerous proteins, and the majority of mitochondria remained intact. In contrast, hypotonic stimuli caused severe damage to mitochondrial structure and function, induced increased oxidative modification of mitochondrial proteins, and brought about detrimental changes to the subproteomes of the inner mitochondrial membrane and matrix. Using an established in vivo murine model of regional myocardial ischemic injury, we validated key observations made by the in vitro model. This preclinical investigation provides function and suborganelle location information on a repertoire of cardiac mitochondrial proteins sensitive to ischemia reperfusion stress and highlights protein clusters potentially involved in mitochondrial dysfunction in the setting of ischemic injury.
Figures
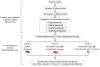
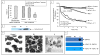
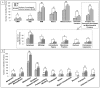
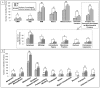
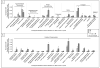
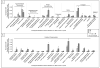
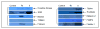
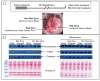
Similar articles
-
MCUB Regulates the Molecular Composition of the Mitochondrial Calcium Uniporter Channel to Limit Mitochondrial Calcium Overload During Stress.Circulation. 2019 Nov 19;140(21):1720-1733. doi: 10.1161/CIRCULATIONAHA.118.037968. Epub 2019 Sep 19. Circulation. 2019. PMID: 31533452 Free PMC article.
-
Oxidative inactivation of key mitochondrial proteins leads to dysfunction and injury in hepatic ischemia reperfusion.Gastroenterology. 2008 Oct;135(4):1344-57. doi: 10.1053/j.gastro.2008.06.048. Epub 2008 Jun 25. Gastroenterology. 2008. PMID: 18778711 Free PMC article.
-
Mitochondrial LonP1 protects cardiomyocytes from ischemia/reperfusion injury in vivo.J Mol Cell Cardiol. 2019 Mar;128:38-50. doi: 10.1016/j.yjmcc.2018.12.017. Epub 2019 Jan 6. J Mol Cell Cardiol. 2019. PMID: 30625302
-
Pathological Roles of Mitochondrial Oxidative Stress and Mitochondrial Dynamics in Cardiac Microvascular Ischemia/Reperfusion Injury.Biomolecules. 2020 Jan 5;10(1):85. doi: 10.3390/biom10010085. Biomolecules. 2020. PMID: 31948043 Free PMC article. Review.
-
Mitochondrial inner membrane lipids and proteins as targets for decreasing cardiac ischemia/reperfusion injury.Pharmacol Ther. 2013 Dec;140(3):258-66. doi: 10.1016/j.pharmthera.2013.07.005. Epub 2013 Jul 15. Pharmacol Ther. 2013. PMID: 23867906 Review.
Cited by
-
Frataxin Deacetylation in Macrophages: Avoiding SIRTain Myocyte Death.Circ Res. 2023 Sep 15;133(7):648-650. doi: 10.1161/CIRCRESAHA.123.323503. Epub 2023 Sep 14. Circ Res. 2023. PMID: 37708247 Free PMC article. No abstract available.
-
Hypoosmosis alters hepatocyte mitochondrial morphology and induces selective release of carbamoyl phosphate synthetase 1.Am J Physiol Gastrointest Liver Physiol. 2023 Oct 1;325(4):G334-G346. doi: 10.1152/ajpgi.00018.2023. Epub 2023 Jul 25. Am J Physiol Gastrointest Liver Physiol. 2023. PMID: 37489865 Free PMC article.
-
Elucidating Mitochondrial Electron Transport Chain Supercomplexes in the Heart During Ischemia-Reperfusion.Antioxid Redox Signal. 2017 Jul 1;27(1):57-69. doi: 10.1089/ars.2016.6635. Epub 2016 Nov 11. Antioxid Redox Signal. 2017. PMID: 27604998 Free PMC article.
-
Duplication of the mitochondrial control region is associated with increased longevity in birds.Aging (Albany NY). 2016 Aug;8(8):1781-9. doi: 10.18632/aging.101012. Aging (Albany NY). 2016. PMID: 27542284 Free PMC article.
-
freeQuant: A Mass Spectrometry Label-Free Quantification Software Tool for Complex Proteome Analysis.ScientificWorldJournal. 2015;2015:137076. doi: 10.1155/2015/137076. Epub 2015 Nov 8. ScientificWorldJournal. 2015. PMID: 26665161 Free PMC article.
References
-
- Lesnefsky EJ, Moghaddas S, Tandler B, Kerner J, Hoppel CL. Mitochondrial dysfunction in cardiac disease ischemia-reperfusion aging and heart failure. J Mol Cell Cardiol. 2001;33:1065–1089. - PubMed
-
- Varadarajan SG, An J, Novalija E, Smart SC, Stowe DF. Changes in [Na(+)](i) compartmental[Ca(2+)] NADH with dysfunction after global ischemia in intact hearts. Am J Physiol. 2001;280:H280–293. - PubMed
-
- Weiss JN, Korge P, Honda HM, Ping P. Role of the mitochondrial permeability transition in myocardial disease. Circ Res. 2003;93:292–301. - PubMed
-
- Honda HM, Korge P, Weiss JN. Mitochondria and ischemia/reperfusion injury. Ann N Y Acad Sci. 2005;1047:248–258. - PubMed
-
- Halestrap A, Calcium P. mitochodria and reperfusion injury: a pore way to die. Biochemical Society Transactions. 2006;34:232–237. - PubMed
Publication types
MeSH terms
Substances
Grants and funding
- R01 HL080691-02/HL/NHLBI NIH HHS/United States
- HL-80691/HL/NHLBI NIH HHS/United States
- HL-80111/HL/NHLBI NIH HHS/United States
- R01 HL065431-02/HL/NHLBI NIH HHS/United States
- P01 HL080111/HL/NHLBI NIH HHS/United States
- R01 HL063901/HL/NHLBI NIH HHS/United States
- R01 HL080691/HL/NHLBI NIH HHS/United States
- P01 HL080111-010002/HL/NHLBI NIH HHS/United States
- F32 HL078109-03/HL/NHLBI NIH HHS/United States
- R37 HL063901/HL/NHLBI NIH HHS/United States
- R01 HL063901-08/HL/NHLBI NIH HHS/United States
- HL-65431/HL/NHLBI NIH HHS/United States
- HL-63901/HL/NHLBI NIH HHS/United States
- R01 HL065431/HL/NHLBI NIH HHS/United States
- SRR 022371-01/PHS HHS/United States
- F32 HL078109/HL/NHLBI NIH HHS/United States
- HL-78109/HL/NHLBI NIH HHS/United States
LinkOut - more resources
Full Text Sources