Detection of low-level promoter activity within open reading frame sequences of Escherichia coli
- PMID: 16260475
- PMCID: PMC1275588
- DOI: 10.1093/nar/gki928
Detection of low-level promoter activity within open reading frame sequences of Escherichia coli
Abstract
The search for promoters has largely been confined to sequences upstream of open reading frames (ORFs) or stable RNA genes. Here we used a cloning approach to discover other potential promoters in Escherichia coli. Chromosomal fragments of approximately 160 bp were fused to a promoterless lacZ reporter gene on a multi-copy plasmid. Eight clones were deliberately selected for high activity and 105 clones were selected at random. All eight of the high-activity clones carried promoters that were located upstream of an ORF. Among the randomly-selected clones, 56 had significantly elevated activity. Of these, 7 had inserts which also mapped upstream of an ORF, while 49 mapped within or downstream of ORFs. Surprisingly, the eight promoters selected for high activity matched the canonical sigma70 -35 and -10 sequences no better than sequences from the randomly-selected clones. For six of the nine most active sequences with orientations opposite to that of the ORF, chromosomal expression was detected by RT-PCR, but defined transcripts were not detected by northern analysis. Our results indicate that the E.coli chromosome carries numerous -35 and -10 sequences with weak promoter activity but that most are not productively expressed because other features needed to enhance promoter activity and transcript stability are absent.
Figures
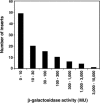
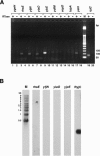
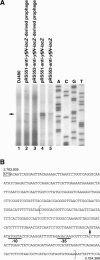
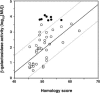
Similar articles
-
Characterization of the hemA-prs region of the Escherichia coli and Salmonella typhimurium chromosomes: identification of two open reading frames and implications for prs expression.J Gen Microbiol. 1993 Feb;139(2):259-66. doi: 10.1099/00221287-139-2-259. J Gen Microbiol. 1993. PMID: 7679718
-
Domain 1.1 of the sigma(70) subunit of Escherichia coli RNA polymerase modulates the formation of stable polymerase/promoter complexes.J Mol Biol. 2001 Jun 8;309(3):561-72. doi: 10.1006/jmbi.2001.4690. J Mol Biol. 2001. PMID: 11397080
-
Identification of a second flagellin gene and functional characterization of a sigma70-like promoter upstream of a Leptospira borgpetersenii flaB gene.Curr Microbiol. 2004 Feb;48(2):145-52. doi: 10.1007/s00284-003-1089-1. Curr Microbiol. 2004. PMID: 15057484
-
Isolation of promoters from two anaerobic bacteria.Microbios. 1988;54(219):87-99. Microbios. 1988. PMID: 2459583
-
Selection of new biological activities from random nucleotide sequences: evolutionary and practical considerations.Genome. 1989;31(1):112-7. doi: 10.1139/g89-021. Genome. 1989. PMID: 2687088 Review.
Cited by
-
Deep Learning-Assisted Design of Novel Promoters in Escherichia coli.Adv Genet (Hoboken). 2023 Nov 15;4(4):2300184. doi: 10.1002/ggn2.202300184. eCollection 2023 Dec. Adv Genet (Hoboken). 2023. PMID: 38099247 Free PMC article.
-
Optimization of Constitutive Promoters Using a Promoter-Trapping Vector in Burkholderia pyrrocinia JK-SH007.Int J Mol Sci. 2023 May 29;24(11):9419. doi: 10.3390/ijms24119419. Int J Mol Sci. 2023. PMID: 37298372 Free PMC article.
-
De Novo Design of the ArsR Regulated Pars Promoter Enables a Highly Sensitive Whole-Cell Biosensor for Arsenic Contamination.Anal Chem. 2022 May 24;94(20):7210-7218. doi: 10.1021/acs.analchem.2c00055. Epub 2022 May 10. Anal Chem. 2022. PMID: 35537205 Free PMC article.
-
Construction of a RFP-lacZα bicistronic reporter system and its application in lead biosensing.PLoS One. 2020 Jan 30;15(1):e0228456. doi: 10.1371/journal.pone.0228456. eCollection 2020. PLoS One. 2020. PMID: 31999769 Free PMC article.
-
Improved Monitoring of Low-Level Transcription in Escherichia coli by a β-Galactosidase α-Complementation System.Front Microbiol. 2019 Jun 26;10:1454. doi: 10.3389/fmicb.2019.01454. eCollection 2019. Front Microbiol. 2019. PMID: 31297105 Free PMC article.
References
-
- Gross C.A., Chan C., Dombroski A., Gruber T., Sharp M., Tupy J., Young B. The functional and regulatory roles of sigma factors in transcription. Cold Spring Harb. Symp. Quant. Biol. 1998;63:141–155. - PubMed
Publication types
MeSH terms
Substances
Grants and funding
LinkOut - more resources
Full Text Sources
Other Literature Sources