Mechanism of positive allosteric modulators acting on AMPA receptors
- PMID: 16192394
- PMCID: PMC6725607
- DOI: 10.1523/JNEUROSCI.2567-05.2005
Mechanism of positive allosteric modulators acting on AMPA receptors
Abstract
Ligand-gated ion channels involved in the modulation of synaptic strength are the AMPA, kainate, and NMDA glutamate receptors. Small molecules that potentiate AMPA receptor currents relieve cognitive deficits caused by neurodegenerative diseases such as Alzheimer's disease and show promise in the treatment of depression. Previously, there has been limited understanding of the molecular mechanism of action for AMPA receptor potentiators. Here we present cocrystal structures of the glutamate receptor GluR2 S1S2 ligand-binding domain in complex with aniracetam [1-(4-methoxybenzoyl)-2-pyrrolidinone] or CX614 (pyrrolidino-1,3-oxazino benzo-1,4-dioxan-10-one), two AMPA receptor potentiators that preferentially slow AMPA receptor deactivation. Both potentiators bind within the dimer interface of the nondesensitized receptor at a common site located on the twofold axis of molecular symmetry. Importantly, the potentiator binding site is adjacent to the "hinge" in the ligand-binding core "clamshell" that undergoes conformational rearrangement after glutamate binding. Using rapid solution exchange, patch-clamp electrophysiology experiments, we show that point mutations of residues that interact with potentiators in the cocrystal disrupt potentiator function. We suggest that the potentiators slow deactivation by stabilizing the clamshell in its closed-cleft, glutamate-bound conformation.
Figures
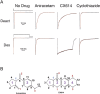
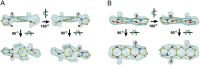
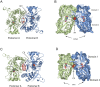
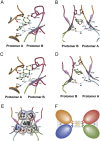
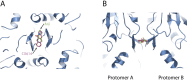
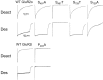
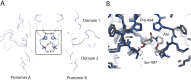
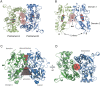
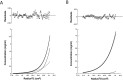
Similar articles
-
Structural and functional analysis of two new positive allosteric modulators of GluA2 desensitization and deactivation.Mol Pharmacol. 2011 Aug;80(2):267-80. doi: 10.1124/mol.110.070243. Epub 2011 May 4. Mol Pharmacol. 2011. PMID: 21543522 Free PMC article.
-
Structural analysis of the positive AMPA receptor modulators CX516 and Me-CX516 in complex with the GluA2 ligand-binding domain.Acta Crystallogr D Biol Crystallogr. 2013 Sep;69(Pt 9):1645-52. doi: 10.1107/S0907444913011839. Epub 2013 Aug 15. Acta Crystallogr D Biol Crystallogr. 2013. PMID: 23999288
-
Novel AMPA receptor potentiators LY392098 and LY404187: effects on recombinant human AMPA receptors in vitro.Neuropharmacology. 2001 Jun;40(8):976-83. doi: 10.1016/s0028-3908(01)00027-2. Neuropharmacology. 2001. PMID: 11406188
-
In search of novel AMPA potentiators.Recent Pat CNS Drug Discov. 2006 Nov;1(3):239-46. doi: 10.2174/157488906778773661. Recent Pat CNS Drug Discov. 2006. PMID: 18221205 Review.
-
AMPA receptor potentiators for the treatment of CNS disorders.Curr Drug Targets CNS Neurol Disord. 2004 Jun;3(3):181-94. doi: 10.2174/1568007043337508. Curr Drug Targets CNS Neurol Disord. 2004. PMID: 15180479 Review.
Cited by
-
Acute ampakines increase voiding function and coordination in a rat model of SCI.Elife. 2024 Mar 7;12:RP89767. doi: 10.7554/eLife.89767. Elife. 2024. PMID: 38451184 Free PMC article.
-
Acute ampakines increase voiding function and coordination in a rat model of SCI.bioRxiv [Preprint]. 2023 Nov 17:2023.05.26.542339. doi: 10.1101/2023.05.26.542339. bioRxiv. 2023. Update in: Elife. 2024 Mar 07;12:RP89767. doi: 10.7554/eLife.89767. PMID: 37293023 Free PMC article. Updated. Preprint.
-
Pharmacological Profile of MP-101, a Novel Non-racemic Mixture of R- and S-dimiracetam with Increased Potency in Rat Models of Cognition, Depression and Neuropathic Pain.Cells. 2022 Dec 13;11(24):4027. doi: 10.3390/cells11244027. Cells. 2022. PMID: 36552791 Free PMC article.
-
Astrocyte: A Foe or a Friend in Intellectual Disability-Related Diseases.Front Synaptic Neurosci. 2022 Jun 23;14:877928. doi: 10.3389/fnsyn.2022.877928. eCollection 2022. Front Synaptic Neurosci. 2022. PMID: 35812794 Free PMC article. Review.
-
Structure, Function, and Pharmacology of Glutamate Receptor Ion Channels.Pharmacol Rev. 2021 Oct;73(4):298-487. doi: 10.1124/pharmrev.120.000131. Pharmacol Rev. 2021. PMID: 34753794 Free PMC article. Review.
References
-
- Arai A, Lynch G (1998) The waveform of synaptic transmission at hippocampal synapses is not determined by AMPA receptor desensitization. Brain Res 799: 230–234. - PubMed
-
- Arai A, Kessler M, Xiao P, Ambros-Ingerson J, Rogers G, Lynch G (1994) A centrally active drug that modulates AMPA receptor gated currents. Brain Res 638: 343–346. - PubMed
-
- Arai A, Kessler M, Ambros-Ingerson J, Quan A, Yigiter E, Rogers G, Lynch G (1996a) Effects of a centrally active benzoylpyrrolidine drug on AMPA receptors kinetics. Neuroscience 75: 573–585. - PubMed
-
- Arai A, Kessler M, Rogers G, Lynch G (1996b) Effects of a memory-enhancing drug on d,l-alpha-amino-3-hydroxy-5-methyl-4-isoxazolepropionic acid receptor currents and synaptic transmission in hippocampus. J Pharmacol Exp Ther 278: 627–638. - PubMed
-
- Arai AC, Kessler M, Rogers G, Lynch G (2000) Effects of the potent ampakine CX614 on hippocampal and recombinant AMPA receptors: interactions with cyclothiazide and GYKI 52466. Mol Pharmacol 58: 802–813. - PubMed
Publication types
MeSH terms
Substances
Grants and funding
LinkOut - more resources
Full Text Sources
Other Literature Sources
Molecular Biology Databases