Staphylococcus aureus RNAIII and the endoribonuclease III coordinately regulate spa gene expression
- PMID: 15678100
- PMCID: PMC549626
- DOI: 10.1038/sj.emboj.7600572
Staphylococcus aureus RNAIII and the endoribonuclease III coordinately regulate spa gene expression
Abstract
Staphylococcus aureus RNAIII is one of the largest regulatory RNAs, which controls several virulence genes encoding exoproteins and cell-wall-associated proteins. One of the RNAIII effects is the repression of spa gene (coding for the surface protein A) expression. Here, we show that spa repression occurs not only at the transcriptional level but also by RNAIII-mediated inhibition of translation and degradation of the stable spa mRNA by the double-strand-specific endoribonuclease III (RNase III). The 3' end domain of RNAIII, partially complementary to the 5' part of spa mRNA, efficiently anneals to spa mRNA through an initial loop-loop interaction. Although this annealing is sufficient to inhibit in vitro the formation of the translation initiation complex, the coordinated action of RNase III is essential in vivo to degrade the mRNA and irreversibly arrest translation. Our results further suggest that RNase III is recruited for targeting the paired RNAs. These findings add further complexity to the expression of the S. aureus virulon.
Figures
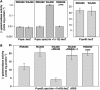
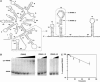
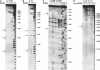
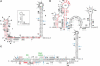




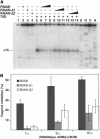
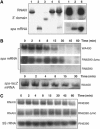
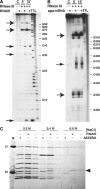


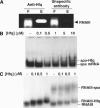
Similar articles
-
Staphylococcus aureus RNAIII coordinately represses the synthesis of virulence factors and the transcription regulator Rot by an antisense mechanism.Genes Dev. 2007 Jun 1;21(11):1353-66. doi: 10.1101/gad.423507. Genes Dev. 2007. PMID: 17545468 Free PMC article.
-
Loop-loop interactions involved in antisense regulation are processed by the endoribonuclease III in Staphylococcus aureus.RNA Biol. 2012 Dec;9(12):1461-72. doi: 10.4161/rna.22710. Epub 2012 Nov 7. RNA Biol. 2012. PMID: 23134978
-
RNAIII activates map expression by forming an RNA-RNA complex in Staphylococcus aureus.FEBS Lett. 2011 Mar 23;585(6):899-905. doi: 10.1016/j.febslet.2011.02.021. Epub 2011 Feb 22. FEBS Lett. 2011. PMID: 21349272
-
Regulatory mechanisms employed by cis-encoded antisense RNAs.Curr Opin Microbiol. 2007 Apr;10(2):102-9. doi: 10.1016/j.mib.2007.03.012. Epub 2007 Mar 26. Curr Opin Microbiol. 2007. PMID: 17387036 Review.
-
Regulation of virulence determinants in Staphylococcus aureus.Int J Med Microbiol. 2001 May;291(2):159-70. doi: 10.1078/1438-4221-00112. Int J Med Microbiol. 2001. PMID: 11437338 Review.
Cited by
-
Unraveling the interplay between a small RNA and RNase E in bacteria.Nucleic Acids Res. 2024 Aug 27;52(15):8947-8966. doi: 10.1093/nar/gkae621. Nucleic Acids Res. 2024. PMID: 39036964 Free PMC article.
-
Chemical and biomolecular insights into the Staphylococcus aureus agr quorum sensing system: Current progress and ongoing challenges.Isr J Chem. 2023 Jun;63(5-6):e202200096. doi: 10.1002/ijch.202200096. Epub 2023 Mar 16. Isr J Chem. 2023. PMID: 38765792 Free PMC article.
-
RNAIII is linked with the pentose phosphate pathway through the activation of RpiRc in Staphylococcus aureus.mSphere. 2024 May 29;9(5):e0034823. doi: 10.1128/msphere.00348-23. Epub 2024 Apr 9. mSphere. 2024. PMID: 38591898 Free PMC article.
-
Staphylococcus aureus foldase PrsA contributes to the folding and secretion of protein A.BMC Microbiol. 2024 Apr 2;24(1):108. doi: 10.1186/s12866-024-03268-7. BMC Microbiol. 2024. PMID: 38566014 Free PMC article.
-
The role of Staphylococcus aureus quorum sensing in cutaneous and systemic infections.Inflamm Regen. 2024 Mar 1;44(1):9. doi: 10.1186/s41232-024-00323-8. Inflamm Regen. 2024. PMID: 38429810 Free PMC article. Review.
References
-
- Argaman L, Altuvia S (2000) fhlA repression by OxyS RNA: kissing complex formation at two sites results in a stable antisense–target RNA complex. J Mol Biol 300: 1101–1112 - PubMed
Publication types
MeSH terms
Substances
LinkOut - more resources
Full Text Sources
Other Literature Sources