Molecular determinants of the inhibition of human Kv1.5 potassium currents by external protons and Zn(2+)
- PMID: 12015417
- PMCID: PMC2290311
- DOI: 10.1113/jphysiol.2001.014456
Molecular determinants of the inhibition of human Kv1.5 potassium currents by external protons and Zn(2+)
Abstract
Using human Kv1.5 channels expressed in HEK293 cells we assessed the ability of H+o to mimic the previously reported action of Zn(2+) to inhibit macroscopic hKv1.5 currents, and using site-directed mutagenesis, we addressed the mechanistic basis for the inhibitory effects of H(+)(o) and Zn(2+). As with Zn(2+), H(+)(o) caused a concentration-dependent, K(+)(o)-sensitive and reversible reduction of the maximum conductance (g(max)). With zero, 5 and 140 mM K(+)(o) the pK(H) for this decrease of g(max) was 6.8, 6.2 and 6.0, respectively. The concentration dependence of the block relief caused by increasing [K(+)](o) was well fitted by a non-competitive interaction between H(+)(o) and K(+)(o), for which the K(D) for the K(+) binding site was 0.5-1.0 mM. Additionally, gating current analysis in the non-conducting mutant hKv1.5 W472F showed that changing from pH 7.4 to pH 5.4 did not affect Q(max) and that charge immobilization, presumed to be due to C-type inactivation, was preserved at pH 5.4. Inhibition of hKv1.5 currents by H+o or Zn(2+) was substantially reduced by a mutation either in the channel turret (H463Q) or near the pore mouth (R487V). In light of the requirement for R487, the homologue of Shaker T449, as well as the block-relieving action of K(+)(o), we propose that H(+) or Zn(2+) binding to histidine residues in the pore turret stabilizes a channel conformation that is most likely an inactivated state.
Figures
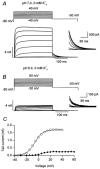
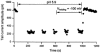
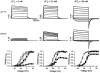
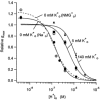
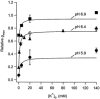
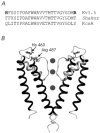
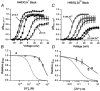
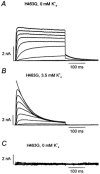
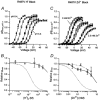
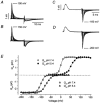
Similar articles
-
Regulation of a mammalian Shaker-related potassium channel, hKv1.5, by extracellular potassium and pH.FEBS Lett. 2001 Jan 12;488(1-2):45-50. doi: 10.1016/s0014-5793(00)02396-6. FEBS Lett. 2001. PMID: 11163793
-
Regulation of transient Na+ conductance by intra- and extracellular K+ in the human delayed rectifier K+ channel Kv1.5.J Physiol. 2000 Mar 15;523 Pt 3(Pt 3):575-91. doi: 10.1111/j.1469-7793.2000.00575.x. J Physiol. 2000. PMID: 10718739 Free PMC article.
-
Regulation of mammalian Shaker-related K+ channels: evidence for non-conducting closed and non-conducting inactivated states.J Physiol. 1998 Jan 15;506 ( Pt 2)(Pt 2):291-301. doi: 10.1111/j.1469-7793.1998.291bw.x. J Physiol. 1998. PMID: 9490854 Free PMC article.
-
External K(+) relieves the block but not the gating shift caused by Zn(2+) in human Kv1.5 potassium channels.J Physiol. 2001 Apr 15;532(Pt 2):349-58. doi: 10.1111/j.1469-7793.2001.0349f.x. J Physiol. 2001. PMID: 11306655 Free PMC article.
-
Synergistic inhibition of the maximum conductance of Kv1.5 channels by extracellular K+ reduction and acidification.Cell Biochem Biophys. 2005;43(2):231-42. doi: 10.1385/CBB:43:2:231. Cell Biochem Biophys. 2005. PMID: 16049348 Review.
Cited by
-
Stability of N-type inactivation and the coupling between N-type and C-type inactivation in the Aplysia Kv1 channel.Pflugers Arch. 2024 Oct;476(10):1493-1516. doi: 10.1007/s00424-024-02982-5. Epub 2024 Jul 15. Pflugers Arch. 2024. PMID: 39008084
-
pH-Channeling in Cancer: How pH-Dependence of Cation Channels Shapes Cancer Pathophysiology.Cancers (Basel). 2020 Sep 2;12(9):2484. doi: 10.3390/cancers12092484. Cancers (Basel). 2020. PMID: 32887220 Free PMC article. Review.
-
Regulation of human cardiac Kv1.5 channels by extracellular acidification.Pflugers Arch. 2016 Nov;468(11-12):1885-1894. doi: 10.1007/s00424-016-1890-x. Epub 2016 Oct 28. Pflugers Arch. 2016. PMID: 27796577
-
The function and regulation of acid-sensing ion channels (ASICs) and the epithelial Na(+) channel (ENaC): IUPHAR Review 19.Br J Pharmacol. 2016 Sep;173(18):2671-701. doi: 10.1111/bph.13533. Epub 2016 Aug 10. Br J Pharmacol. 2016. PMID: 27278329 Free PMC article. Review.
-
Putative binding sites for arachidonic acid on the human cardiac Kv 1.5 channel.Br J Pharmacol. 2015 Nov;172(22):5281-92. doi: 10.1111/bph.13314. Epub 2015 Oct 22. Br J Pharmacol. 2015. PMID: 26292661 Free PMC article.
References
-
- Aiyar J, Withka JM, Rizzi JP, Singleton DH, Andrews GC, Lin W, Boyd J, Hanson DC, Simon M, Dethlefs B. Topology of the pore-region of a K+ channel revealed by the NMR-derived structures of scorpion toxins. Neuron. 1995;15:1169–1181. - PubMed
-
- Backx PH, Yue DT, Lawrence JH, Marban E, Tomaselli GF. Molecular localization of an ion-binding site within the pore of mammalian sodium channels. Science. 1992;257:248–251. - PubMed
-
- Baukrowitz T, Yellen G. Modulation of K+ current by frequency and external [K+]: A tale of two inactivation mechanisms. Neuron. 1995;15:951–960. - PubMed
-
- Blaustein RO, Cole PA, Williams C, Miller C. Tethered blockers as molecular ‘tape measures’ for a voltage-gated K+ channel. Nature Structural Biology. 2000;7:309–311. - PubMed
Publication types
MeSH terms
Substances
LinkOut - more resources
Full Text Sources
Research Materials