Cardiac-derived extracellular vesicles improve mitochondrial function to protect the heart against ischemia/reperfusion injury by delivering ATP5a1
- PMID: 38951822
- PMCID: PMC11218245
- DOI: 10.1186/s12951-024-02618-x
Cardiac-derived extracellular vesicles improve mitochondrial function to protect the heart against ischemia/reperfusion injury by delivering ATP5a1
Abstract
Background: Numerous studies have confirmed the involvement of extracellular vesicles (EVs) in various physiological processes, including cellular death and tissue damage. Recently, we reported that EVs derived from ischemia-reperfusion heart exacerbate cardiac injury. However, the role of EVs from healthy heart tissue (heart-derived EVs, or cEVs) on myocardial ischemia-reperfusion (MI/R) injury remains unclear.
Results: Here, we demonstrated that intramyocardial administration of cEVs significantly enhanced cardiac function and reduced cardiac damage in murine MI/R injury models. cEVs treatment effectively inhibited ferroptosis and maintained mitochondrial homeostasis in cardiomyocytes subjected to ischemia-reperfusion injury. Further results revealed that cEVs can transfer ATP5a1 into cardiomyocytes, thereby suppressing mitochondrial ROS production, alleviating mitochondrial damage, and inhibiting cardiomyocyte ferroptosis. Knockdown of ATP5a1 abolished the protective effects of cEVs. Furthermore, we found that the majority of cEVs are derived from cardiomyocytes, and ATP5a1 in cEVs primarily originates from cardiomyocytes of the healthy murine heart. Moreover, we demonstrated that adipose-derived stem cells (ADSC)-derived EVs with ATP5a1 overexpression showed much better efficacy on the therapy of MI/R injury compared to control ADSC-derived EVs.
Conclusions: These findings emphasized the protective role of cEVs in cardiac injury and highlighted the therapeutic potential of targeting ATP5a1 as an important approach for managing myocardial damage induced by MI/R injury.
Keywords: ATP5a1; Extracellular vesicles; Ferroptosis; Mitochondria; Myocardial ischemia-reperfusion.
© 2024. The Author(s).
Conflict of interest statement
The authors declare no competing interests.
Figures
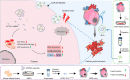
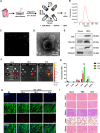
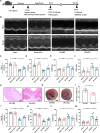
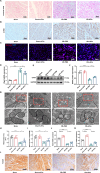
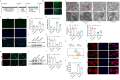
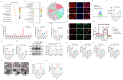
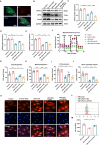
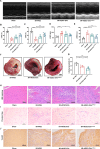
Similar articles
-
Extracellular vesicles derived from different tissues attenuate cardiac dysfunction in murine MI models.Biol Direct. 2023 Nov 17;18(1):76. doi: 10.1186/s13062-023-00429-y. Biol Direct. 2023. PMID: 37978390 Free PMC article.
-
Deletion of capn4 Protects the Heart Against Endotoxemic Injury by Preventing ATP Synthase Disruption and Inhibiting Mitochondrial Superoxide Generation.Circ Heart Fail. 2015 Sep;8(5):988-96. doi: 10.1161/CIRCHEARTFAILURE.115.002383. Epub 2015 Aug 5. Circ Heart Fail. 2015. PMID: 26246018 Free PMC article.
-
Activation of PPAR-α attenuates myocardial ischemia/reperfusion injury by inhibiting ferroptosis and mitochondrial injury via upregulating 14-3-3η.Sci Rep. 2024 Jul 2;14(1):15246. doi: 10.1038/s41598-024-64638-9. Sci Rep. 2024. PMID: 38956068 Free PMC article.
-
Protective effects of natural products against myocardial ischemia/reperfusion: Mitochondria-targeted therapeutics.Biomed Pharmacother. 2022 May;149:112893. doi: 10.1016/j.biopha.2022.112893. Epub 2022 Mar 31. Biomed Pharmacother. 2022. PMID: 35366532 Review.
-
Regulation of metabolism by mitochondrial enzyme acetylation in cardiac ischemia-reperfusion injury.Biochim Biophys Acta Mol Basis Dis. 2020 Jun 1;1866(6):165728. doi: 10.1016/j.bbadis.2020.165728. Epub 2020 Feb 15. Biochim Biophys Acta Mol Basis Dis. 2020. PMID: 32068115 Free PMC article. Review.
Cited by
-
Nuclear receptor subfamily 4 group A member 1 promotes myocardial ischemia/reperfusion injury through inducing mitochondrial fission factor-mediated mitochondrial fragmentation and inhibiting FUN14 domain containing 1-depedent mitophagy.Int J Biol Sci. 2024 Aug 19;20(11):4458-4475. doi: 10.7150/ijbs.95853. eCollection 2024. Int J Biol Sci. 2024. PMID: 39247823 Free PMC article.
-
Hepatocyte-derived tissue extracellular vesicles safeguard liver regeneration and support regenerative therapy.J Nanobiotechnology. 2024 Aug 30;22(1):521. doi: 10.1186/s12951-024-02790-0. J Nanobiotechnology. 2024. PMID: 39210346 Free PMC article.
References
-
- Heusch G, Gersh BJ. The pathophysiology of acute myocardial infarction and strategies of protection beyond reperfusion: a continual challenge. Eur Heart J. 2017;38(11):774–84. - PubMed
MeSH terms
Substances
Grants and funding
- PWZxk-2022-20/the Key Discipline Construction Project of Shanghai Pudong New Area Health Commission
- 23ZR1452500/the Program of Science and Technology Commission of Shanghai Municipality
- 81670458/the National Natural Science Foundation of China
- 23ZR1452500/the program of Science and Technology Commission of Shanghai Municipality
LinkOut - more resources
Full Text Sources
Miscellaneous