Selective processing of all rotational and translational optic flow directions in the zebrafish pretectum and tectum
- PMID: 30925897
- PMCID: PMC6441171
- DOI: 10.1186/s12915-019-0648-2
Selective processing of all rotational and translational optic flow directions in the zebrafish pretectum and tectum
Abstract
Background: The processing of optic flow in the pretectum/accessory optic system allows animals to stabilize retinal images by executing compensatory optokinetic and optomotor behavior. The success of this behavior depends on the integration of information from both eyes to unequivocally identify all possible translational or rotational directions of motion. However, it is still unknown whether the precise direction of ego-motion is already identified in the zebrafish pretectum or later in downstream premotor areas.
Results: Here, we show that the zebrafish pretectum and tectum each contain four populations of motion-sensitive direction-selective (DS) neurons, with each population encoding a different preferred direction upon monocular stimulation. In contrast, binocular stimulation revealed the existence of pretectal and tectal neurons that are specifically tuned to only one of the many possible combinations of monocular motion, suggesting that further downstream sensory processing might not be needed to instruct appropriate optokinetic and optomotor behavior.
Conclusion: Our results suggest that local, task-specific pretectal circuits process DS retinal inputs and carry out the binocular sensory computations necessary for optokinetic and optomotor behavior.
Keywords: Binocular integration; Calcium imaging; Optic flow; Optic tectum; Optokinetic response; Optomotor response; Pretectum; Zebrafish.
Conflict of interest statement
Ethics approval and consent to participate
The animal experiments were licensed by the local authorities (Regierungspräsidium Tübingen) in accordance with German federal law and Baden-Württemberg state law.
Competing interests
All authors declare that they have no competing interests.
Publisher’s Note
Springer Nature remains neutral with regard to jurisdictional claims in published maps and institutional affiliations.
Figures
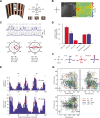
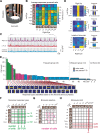
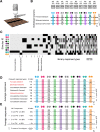
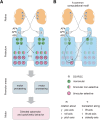
Similar articles
-
Neuronal Architecture of a Visual Center that Processes Optic Flow.Neuron. 2019 Jul 3;103(1):118-132.e7. doi: 10.1016/j.neuron.2019.04.018. Epub 2019 May 27. Neuron. 2019. PMID: 31147153
-
Parallel Channels for Motion Feature Extraction in the Pretectum and Tectum of Larval Zebrafish.Cell Rep. 2020 Jan 14;30(2):442-453.e6. doi: 10.1016/j.celrep.2019.12.031. Cell Rep. 2020. PMID: 31940488
-
Circuit Organization Underlying Optic Flow Processing in Zebrafish.Front Neural Circuits. 2021 Jul 21;15:709048. doi: 10.3389/fncir.2021.709048. eCollection 2021. Front Neural Circuits. 2021. PMID: 34366797 Free PMC article. Review.
-
A robust receptive field code for optic flow detection and decomposition during self-motion.Curr Biol. 2022 Jun 6;32(11):2505-2516.e8. doi: 10.1016/j.cub.2022.04.048. Epub 2022 May 11. Curr Biol. 2022. PMID: 35550724
-
Direction selectivity in the visual system of the zebrafish larva.Front Neural Circuits. 2013 Jun 18;7:111. doi: 10.3389/fncir.2013.00111. eCollection 2013. Front Neural Circuits. 2013. PMID: 23785314 Free PMC article. Review.
Cited by
-
Transcriptional control of visual neural circuit development by GS homeobox 1.PLoS Genet. 2024 Apr 26;20(4):e1011139. doi: 10.1371/journal.pgen.1011139. eCollection 2024 Apr. PLoS Genet. 2024. PMID: 38669217 Free PMC article.
-
Zebrafish Optokinetic Reflex: Minimal Reporting Guidelines and Recommendations.Biology (Basel). 2023 Dec 20;13(1):4. doi: 10.3390/biology13010004. Biology (Basel). 2023. PMID: 38275725 Free PMC article. Review.
-
Structural and Functional Organization of Visual Responses in the Inferior Olive of Larval Zebrafish.J Neurosci. 2024 Feb 21;44(8):e2352212023. doi: 10.1523/JNEUROSCI.2352-21.2023. J Neurosci. 2024. PMID: 38195508 Free PMC article.
-
A behavioral and modeling study of control algorithms underlying the translational optomotor response in larval zebrafish with implications for neural circuit function.PLoS Comput Biol. 2023 Feb 23;19(2):e1010924. doi: 10.1371/journal.pcbi.1010924. eCollection 2023 Feb. PLoS Comput Biol. 2023. PMID: 36821587 Free PMC article.
-
Optic flow in the natural habitats of zebrafish supports spatial biases in visual self-motion estimation.Curr Biol. 2022 Dec 5;32(23):5008-5021.e8. doi: 10.1016/j.cub.2022.10.009. Epub 2022 Nov 2. Curr Biol. 2022. PMID: 36327979 Free PMC article.
References
-
- Masseck OA, Hoffmann KP. Comparative neurobiology of the optokinetic reflex. Ann N Y Acad Sci. 2009;1164:430–439. - PubMed
-
- Orger MB, Gahtan E, Muto A, Page-McCaw P, Smear MC, Baier H. Behavioral screening assays in zebrafish. Methods Cell Biol. 2004;77:53–68. - PubMed
-
- Maaswinkel H, Li L. Spatio-temporal frequency characteristics of the optomotor response in zebrafish. Vis Res. 2003;43(1):21–30. - PubMed
-
- Busch C, Borst A, Mauss AS. Bi-directional control of walking behavior by horizontal optic flow sensors. Curr Biol. 2018;28(24):4037–45 e5. - PubMed
Publication types
MeSH terms
LinkOut - more resources
Full Text Sources
Molecular Biology Databases