Biochemical and structural analyses of a bacterial endo-β-1,2-glucanase reveal a new glycoside hydrolase family
- PMID: 28270506
- PMCID: PMC5418048
- DOI: 10.1074/jbc.M116.762724
Biochemical and structural analyses of a bacterial endo-β-1,2-glucanase reveal a new glycoside hydrolase family
Abstract
β-1,2-Glucan is an extracellular cyclic or linear polysaccharide from Gram-negative bacteria, with important roles in infection and symbiosis. Despite β-1,2-glucan's importance in bacterial persistence and pathogenesis, only a few reports exist on enzymes acting on both cyclic and linear β-1,2-glucan. To this end, we purified an endo-β-1,2-glucanase to homogeneity from cell extracts of the environmental species Chitinophaga arvensicola, and an endo-β-1,2-glucanase candidate gene (Cpin_6279) was cloned from the related species Chitinophaga pinensis The Cpin_6279 protein specifically hydrolyzed linear β-1,2-glucan with polymerization degrees of ≥5 and a cyclic counterpart, indicating that Cpin_6279 is an endo-β-1,2-glucananase. Stereochemical analysis demonstrated that the Cpin_6279-catalyzed reaction proceeds via an inverting mechanism. Cpin_6279 exhibited no significant sequence similarity with known glycoside hydrolases (GHs), and thus the enzyme defines a novel GH family, GH144. The crystal structures of the ligand-free and complex forms of Cpin_6279 with glucose (Glc) and sophorotriose (Glc-β-1,2-Glc-β-1,2-Glc) determined up to 1.7 Å revealed that it has a large cavity appropriate for polysaccharide degradation and adopts an (α/α)6-fold slightly similar to that of GH family 15 and 8 enzymes. Mutational analysis indicated that some of the highly conserved acidic residues in the active site are important for catalysis, and the Cpin_6279 active-site architecture provided insights into the substrate recognition by the enzyme. The biochemical characterization and crystal structure of this novel GH may enable discovery of other β-1,2-glucanases and represent a critical advance toward elucidating structure-function relationships of GH enzymes.
Keywords: Chitinophaga pinensis; Gram-negative bacteria; endo-β-1,2-glucanase; enzyme structure; glycosidase; novel glycoside hydrolase family; oligosaccharide; polysaccharide; sophorooligosaccharide; β-1,2-glucan.
© 2017 by The American Society for Biochemistry and Molecular Biology, Inc.
Conflict of interest statement
The authors declare that they have no conflicts of interest with the contents of this article
Figures
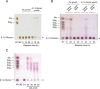
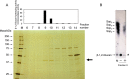
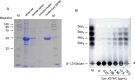
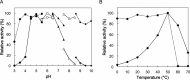
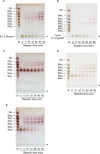
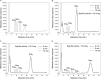
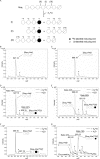
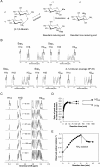
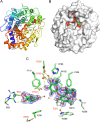
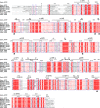
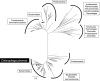
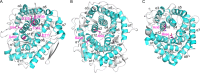
Similar articles
-
Identification, characterization, and structural analyses of a fungal endo-β-1,2-glucanase reveal a new glycoside hydrolase family.J Biol Chem. 2019 May 10;294(19):7942-7965. doi: 10.1074/jbc.RA118.007087. Epub 2019 Mar 29. J Biol Chem. 2019. PMID: 30926603 Free PMC article.
-
Structural insights into substrate recognition and catalysis by glycoside hydrolase family 87 α-1,3-glucanase from Paenibacillus glycanilyticus FH11.FEBS J. 2020 Jun;287(12):2524-2543. doi: 10.1111/febs.15161. Epub 2019 Dec 19. FEBS J. 2020. PMID: 31788942
-
Structure-Function Analysis of a Mixed-linkage β-Glucanase/Xyloglucanase from the Key Ruminal Bacteroidetes Prevotella bryantii B(1)4.J Biol Chem. 2016 Jan 15;291(3):1175-97. doi: 10.1074/jbc.M115.691659. Epub 2015 Oct 27. J Biol Chem. 2016. PMID: 26507654 Free PMC article.
-
Review: The structure and function of cellulase (endo-β-1,4-glucanase) and hemicellulase (β-1,3-glucanase and endo-β-1,4-mannase) enzymes in invertebrates that consume materials ranging from microbes, algae to leaf litter.Comp Biochem Physiol B Biochem Mol Biol. 2020 Feb;240:110354. doi: 10.1016/j.cbpb.2019.110354. Epub 2019 Oct 21. Comp Biochem Physiol B Biochem Mol Biol. 2020. PMID: 31647988 Review.
-
Bacterial α-Glucan and Branching Sucrases from GH70 Family: Discovery, Structure-Function Relationship Studies and Engineering.Microorganisms. 2021 Jul 28;9(8):1607. doi: 10.3390/microorganisms9081607. Microorganisms. 2021. PMID: 34442685 Free PMC article. Review.
Cited by
-
Discovery of Anomer-Inverting Transglycosylase: Cyclic Glucohexadecaose-Producing Enzyme from Xanthomonas, a Phytopathogen.J Am Chem Soc. 2024 Jul 3;146(26):17738-17746. doi: 10.1021/jacs.4c02579. Epub 2024 Jun 19. J Am Chem Soc. 2024. PMID: 38957137 Free PMC article.
-
Structural and biochemical analysis of family 92 carbohydrate-binding modules uncovers multivalent binding to β-glucans.Nat Commun. 2024 Apr 23;15(1):3429. doi: 10.1038/s41467-024-47584-y. Nat Commun. 2024. PMID: 38653764 Free PMC article.
-
Structure-function analysis of the cyclic β-1,2-glucan synthase from Agrobacterium tumefaciens.Nat Commun. 2024 Feb 28;15(1):1844. doi: 10.1038/s41467-024-45415-8. Nat Commun. 2024. PMID: 38418509 Free PMC article.
-
Functional and structural analysis of a cyclization domain in a cyclic β-1,2-glucan synthase.Appl Microbiol Biotechnol. 2024 Feb 1;108(1):187. doi: 10.1007/s00253-024-13013-9. Appl Microbiol Biotechnol. 2024. PMID: 38300345 Free PMC article.
-
Identification of enzymatic functions of osmo-regulated periplasmic glucan biosynthesis proteins from Escherichia coli reveals a novel glycoside hydrolase family.Commun Biol. 2023 Sep 21;6(1):961. doi: 10.1038/s42003-023-05336-6. Commun Biol. 2023. PMID: 37735577 Free PMC article.
References
Publication types
MeSH terms
Substances
Associated data
- Actions
- Actions
- Actions
- Actions
- Actions
- Actions
- Actions
LinkOut - more resources
Full Text Sources
Other Literature Sources
Research Materials