Perturbation of m6A writers reveals two distinct classes of mRNA methylation at internal and 5' sites
- PMID: 24981863
- PMCID: PMC4142486
- DOI: 10.1016/j.celrep.2014.05.048
Perturbation of m6A writers reveals two distinct classes of mRNA methylation at internal and 5' sites
Abstract
N6-methyladenosine (m6A) is a common modification of mRNA with potential roles in fine-tuning the RNA life cycle. Here, we identify a dense network of proteins interacting with METTL3, a component of the methyltransferase complex, and show that three of them (WTAP, METTL14, and KIAA1429) are required for methylation. Monitoring m6A levels upon WTAP depletion allowed the definition of accurate and near single-nucleotide resolution methylation maps and their classification into WTAP-dependent and -independent sites. WTAP-dependent sites are located at internal positions in transcripts, topologically static across a variety of systems we surveyed, and inversely correlated with mRNA stability, consistent with a role in establishing "basal" degradation rates. WTAP-independent sites form at the first transcribed base as part of the cap structure and are present at thousands of sites, forming a previously unappreciated layer of transcriptome complexity. Our data shed light on the proteomic and transcriptional underpinnings of this RNA modification.
Copyright © 2014 The Authors. Published by Elsevier Inc. All rights reserved.
Figures
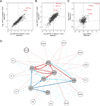
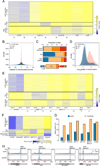
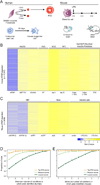
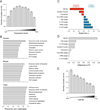
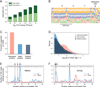
Similar articles
-
METTL3 regulates m6A in endometrioid epithelial ovarian cancer independently of METTl14 and WTAP.Cell Biol Int. 2020 Dec;44(12):2524-2531. doi: 10.1002/cbin.11459. Epub 2020 Sep 11. Cell Biol Int. 2020. PMID: 32869897
-
Mammalian WTAP is a regulatory subunit of the RNA N6-methyladenosine methyltransferase.Cell Res. 2014 Feb;24(2):177-89. doi: 10.1038/cr.2014.3. Epub 2014 Jan 10. Cell Res. 2014. PMID: 24407421 Free PMC article.
-
Interactions, localization, and phosphorylation of the m6A generating METTL3-METTL14-WTAP complex.RNA. 2018 Apr;24(4):499-512. doi: 10.1261/rna.064063.117. Epub 2018 Jan 18. RNA. 2018. PMID: 29348140 Free PMC article.
-
The Functions of N-methyladenosine (m6A) Modification on HIV-1 mRNA.Cell Biochem Biophys. 2024 Jun;82(2):561-574. doi: 10.1007/s12013-024-01280-2. Epub 2024 May 16. Cell Biochem Biophys. 2024. PMID: 38753251 Review.
-
RNA N6-methyladenosine methylation in post-transcriptional gene expression regulation.Genes Dev. 2015 Jul 1;29(13):1343-55. doi: 10.1101/gad.262766.115. Genes Dev. 2015. PMID: 26159994 Free PMC article. Review.
Cited by
-
Global Co-regulatory Cross Talk Between m6A and m5C RNA Methylation Systems Coordinate Cellular Responses and Brain Disease Pathways.Mol Neurobiol. 2024 Nov 5. doi: 10.1007/s12035-024-04555-0. Online ahead of print. Mol Neurobiol. 2024. PMID: 39499421
-
Trends and frontiers of RNA methylation in cancer over the past 10 years: a bibliometric and visual analysis.Front Genet. 2024 Oct 14;15:1461386. doi: 10.3389/fgene.2024.1461386. eCollection 2024. Front Genet. 2024. PMID: 39473440 Free PMC article.
-
Interplay of RNA m6A Modification-Related Geneset in Pan-Cancer.Biomedicines. 2024 Sep 27;12(10):2211. doi: 10.3390/biomedicines12102211. Biomedicines. 2024. PMID: 39457524 Free PMC article.
-
N6-Methyladenosine Methyltransferase Component KIAA1429 Is a Potential Target of Cancer Therapy.Biomolecules. 2024 Oct 17;14(10):1319. doi: 10.3390/biom14101319. Biomolecules. 2024. PMID: 39456252 Free PMC article. Review.
-
Decoding the epitranscriptome: a new frontier for cancer therapy and drug resistance.Cell Commun Signal. 2024 Oct 21;22(1):513. doi: 10.1186/s12964-024-01854-w. Cell Commun Signal. 2024. PMID: 39434167 Free PMC article. Review.
References
-
- Bembom O. seqLogo: Sequence logos for DNA sequence alignments. 2011
-
- Bokar J, Rath-Shambaugh M, Ludwiczak R, Narayan P, Rottman F. Characterization and partial purification of mRNA N6-adenosine methyltransferase from HeLa cell nuclei. Internal mRNA methylation requires a multisubunit complex. The Journal of biological chemistry. 1994;269:17697–17704. - PubMed
-
- Bokar JA. The biosynthesis and functional roles of methylated nucleosides in eukaryotic mRNA. In: Grosjean Henri., editor. Fine-Tuning of RNA Functions by Modification and Editing. Vol. 12. 2005. pp. 141–177.
Publication types
MeSH terms
Substances
Grants and funding
LinkOut - more resources
Full Text Sources
Other Literature Sources
Molecular Biology Databases
Miscellaneous