Bacterial wall as target for attack: past, present, and future research
- PMID: 14557293
- PMCID: PMC207114
- DOI: 10.1128/CMR.16.4.673-687.2003
Bacterial wall as target for attack: past, present, and future research
Abstract
When Bacteria, Archaea, and Eucarya separated from each other, a great deal of evolution had taken place. Only then did extensive diversity arise. The bacteria split off with the new property that they had a sacculus that protected them from their own turgor pressure. The saccular wall of murein (or peptidoglycan) was an effective solution to the osmotic pressure problem, but it then was a target for other life-forms, which created lysoymes and beta-lactams. The beta-lactams, with their four-member strained rings, are effective agents in nature and became the first antibiotic in human medicine. But that is by no means the end of the story. Over evolutionary time, bacteria challenged by beta-lactams evolved countermeasures such as beta-lactamases, and the producing organisms evolved variant beta-lactams. The biology of both classes became evident as the pharmaceutical industry isolated, modified, and produced new chemotherapeutic agents and as the properties of beta-lactams and beta-lactamases were examined by molecular techniques. This review attempts to fit the wall biology of current microbes and their clinical context into the way organisms developed on this planet as well as the changes arising since the work done by Fleming. It also outlines the scientific advances in our understanding of this broad area of biology.
Figures
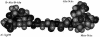
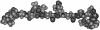
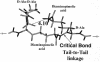
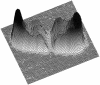
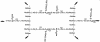
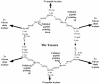
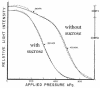
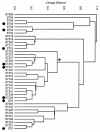
Similar articles
-
The bacterial cell wall as a source of antibacterial targets.Expert Opin Ther Targets. 2002 Feb;6(1):1-19. doi: 10.1517/14728222.6.1.1. Expert Opin Ther Targets. 2002. PMID: 11901475 Review.
-
Antibiotic resistance in pathogenic and producing bacteria, with special reference to beta-lactam antibiotics.Microbiol Rev. 1981 Dec;45(4):591-619. doi: 10.1128/mr.45.4.591-619.1981. Microbiol Rev. 1981. PMID: 7035856 Free PMC article. Review. No abstract available.
-
Constructing and deconstructing the bacterial cell wall.Protein Sci. 2020 Mar;29(3):629-646. doi: 10.1002/pro.3737. Epub 2019 Nov 20. Protein Sci. 2020. PMID: 31747090 Free PMC article. Review.
-
Beta-lactam antibiotics: from antibiosis to resistance and bacteriology.APMIS. 2010 Jan;118(1):1-36. doi: 10.1111/j.1600-0463.2009.02563.x. APMIS. 2010. PMID: 20041868 Free PMC article. Review.
-
Future chemotherapy, with emphasis on bacterial murein.FEMS Immunol Med Microbiol. 2006 Mar;46(2):158-65. doi: 10.1111/j.1574-695X.2005.00032.x. FEMS Immunol Med Microbiol. 2006. PMID: 16487296 Review. No abstract available.
Cited by
-
Assessment of the Salivary Concentrations of Selected Immunological Components in Adult Patients in the Late Period after Allogeneic Hematopoietic Stem Cell Transplantation-A Translational Study.Int J Mol Sci. 2024 Jan 25;25(3):1457. doi: 10.3390/ijms25031457. Int J Mol Sci. 2024. PMID: 38338734 Free PMC article.
-
Identification of isothiazolones analogues as potent bactericidal agents against antibiotic resistant CRE and MRSA strains.BMC Chem. 2023 Dec 16;17(1):183. doi: 10.1186/s13065-023-01100-3. BMC Chem. 2023. PMID: 38104171 Free PMC article.
-
Study of novel bidentate heterocyclic amine-based metal complexes and their biological activities: cytotoxicity and antimicrobial activity evaluation.BMC Chem. 2023 Jul 15;17(1):78. doi: 10.1186/s13065-023-00996-1. BMC Chem. 2023. PMID: 37454081 Free PMC article.
-
Exploring antibiotic resistance with chemical tools.Chem Commun (Camb). 2023 May 18;59(41):6148-6158. doi: 10.1039/d3cc00759f. Chem Commun (Camb). 2023. PMID: 37039397 Free PMC article. Review.
-
Antimicrobial Peptides-Mechanisms of Action, Antimicrobial Effects and Clinical Applications.Antibiotics (Basel). 2022 Oct 16;11(10):1417. doi: 10.3390/antibiotics11101417. Antibiotics (Basel). 2022. PMID: 36290075 Free PMC article. Review.
References
-
- Abramson, E. P., and E. Chain. 1940. An enzyme from bacteria able to destroy penicillin. Nature 146:837. - PubMed
-
- Barlow, M., and B. G. Hall. 2002. Phylogenetic analysis shows that OXA β-lactamase genes have been on plasmids for millions of years. J. Mol. Evol. 55:314-321. - PubMed
-
- Brown, J. T. R., C. J. Douady, M. J. Italia, W. E. Marshall, and M. J. Stanhope. 2001. Universal trees based on large combined protein sequence data sets. Nat. Genet. 28:281-285. - PubMed
-
- Bush, K. 1999. β-Lactamases of increasing clinical importance. Curr. Pharm. Design 5:839-845. - PubMed
-
- Bush, K. 2001. New beta-lactamases in gram-negative bacteria: diversity and impact on the selection of antimicrobial therapy. Clin. Infect. Dis. 32:1085-1089. - PubMed
Publication types
MeSH terms
Substances
LinkOut - more resources
Full Text Sources
Other Literature Sources
Medical
Miscellaneous