Evolution of translational control and the emergence of genes and open reading frames in human and non-human primate hearts
- PMID: 39317836
- PMCID: PMC11473369
- DOI: 10.1038/s44161-024-00544-7
Evolution of translational control and the emergence of genes and open reading frames in human and non-human primate hearts
Abstract
Evolutionary innovations can be driven by changes in the rates of RNA translation and the emergence of new genes and small open reading frames (sORFs). In this study, we characterized the transcriptional and translational landscape of the hearts of four primate and two rodent species through integrative ribosome and transcriptomic profiling, including adult left ventricle tissues and induced pluripotent stem cell-derived cardiomyocyte cell cultures. We show here that the translational efficiencies of subunits of the mitochondrial oxidative phosphorylation chain complexes IV and V evolved rapidly across mammalian evolution. Moreover, we discovered hundreds of species-specific and lineage-specific genomic innovations that emerged during primate evolution in the heart, including 551 genes, 504 sORFs and 76 evolutionarily conserved genes displaying human-specific cardiac-enriched expression. Overall, our work describes the evolutionary processes and mechanisms that have shaped cardiac transcription and translation in recent primate evolution and sheds light on how these can contribute to cardiac development and disease.
© 2024. The Author(s).
Conflict of interest statement
The authors declare no competing interests.
Figures
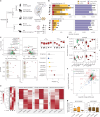
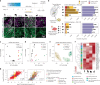
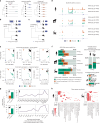
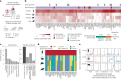
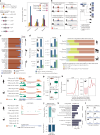
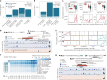
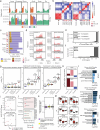
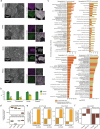
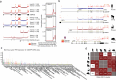
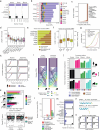
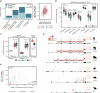
Similar articles
-
Discovery of Unannotated Small Open Reading Frames in Streptococcus pneumoniae D39 Involved in Quorum Sensing and Virulence Using Ribosome Profiling.mBio. 2022 Aug 30;13(4):e0124722. doi: 10.1128/mbio.01247-22. Epub 2022 Jul 19. mBio. 2022. PMID: 35852327 Free PMC article.
-
The cardiac translational landscape reveals that micropeptides are new players involved in cardiomyocyte hypertrophy.Mol Ther. 2021 Jul 7;29(7):2253-2267. doi: 10.1016/j.ymthe.2021.03.004. Epub 2021 Mar 5. Mol Ther. 2021. PMID: 33677093 Free PMC article.
-
Ribosome Profiling and RNA Sequencing Reveal Translation and Transcription Regulation under Acute Heat Stress in Rainbow Trout (Oncorhynchus mykiss, Walbaum, 1792) Liver.Int J Mol Sci. 2024 Aug 14;25(16):8848. doi: 10.3390/ijms25168848. Int J Mol Sci. 2024. PMID: 39201531 Free PMC article.
-
Evolution of new proteins from translated sORFs in long non-coding RNAs.Exp Cell Res. 2020 Jun 1;391(1):111940. doi: 10.1016/j.yexcr.2020.111940. Epub 2020 Mar 7. Exp Cell Res. 2020. PMID: 32156600 Review.
-
Translation of Small Open Reading Frames: Roles in Regulation and Evolutionary Innovation.Trends Genet. 2019 Mar;35(3):186-198. doi: 10.1016/j.tig.2018.12.003. Epub 2018 Dec 31. Trends Genet. 2019. PMID: 30606460 Review.
References
-
- Marlowe, F. W. Hunter-gatherers and human evolution. Evol. Anthropol.14, 54–67 (2005).
-
- Lowenstine, L. J., McManamon, R. & Terio, K. A. Comparative pathology of aging great apes: bonobos, chimpanzees, gorillas, and orangutans. Vet. Pathol.53, 250–276 (2016). - PubMed
Publication types
MeSH terms
LinkOut - more resources
Full Text Sources