A sequence of SVA retrotransposon insertions in ASIP shaped human pigmentation
- PMID: 39048794
- PMCID: PMC11319198
- DOI: 10.1038/s41588-024-01841-4
A sequence of SVA retrotransposon insertions in ASIP shaped human pigmentation
Abstract
Retrotransposons comprise about 45% of the human genome1, but their contributions to human trait variation and evolution are only beginning to be explored2,3. Here, we find that a sequence of SVA retrotransposon insertions in an early intron of the ASIP (agouti signaling protein) gene has probably shaped human pigmentation several times. In the UK Biobank (n = 169,641), a recent 3.3-kb SVA insertion polymorphism associated strongly with lighter skin pigmentation (0.22 [0.21-0.23] s.d.; P = 2.8 × 10-351) and increased skin cancer risk (odds ratio = 1.23 [1.18-1.27]; P = 1.3 × 10-28), appearing to underlie one of the strongest common genetic influences on these phenotypes within European populations4-6. ASIP expression in skin displayed the same association pattern, with the SVA insertion allele exhibiting 2.2-fold (1.9-2.6) increased expression. This effect had an unusual apparent mechanism: an earlier, nonpolymorphic, human-specific SVA retrotransposon 3.9 kb upstream appeared to have caused ASIP hypofunction by nonproductive splicing, which the new (polymorphic) SVA insertion largely eliminated. Extended haplotype homozygosity indicated that the insertion allele has risen to allele frequencies up to 11% in European populations over the past several thousand years. These results indicate that a sequence of retrotransposon insertions contributed to a species-wide increase, then a local decrease, of human pigmentation.
© 2024. The Author(s).
Conflict of interest statement
The authors declare no competing interests.
Figures
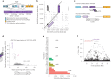
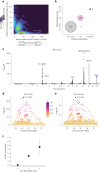
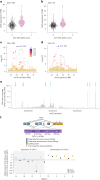
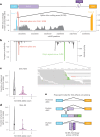
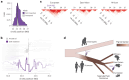
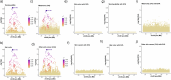
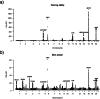
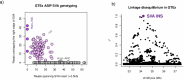
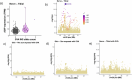
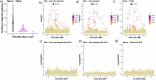
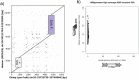
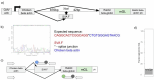
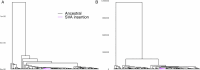
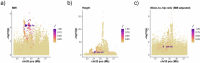
Similar articles
-
Long-read sequencing identifies an SVA_D retrotransposon insertion deep within the intron of ATP7A as a novel cause of occipital horn syndrome.J Med Genet. 2024 Sep 24;61(10):950-958. doi: 10.1136/jmg-2024-110056. J Med Genet. 2024. PMID: 38960580
-
Genetic variants in pigmentation genes, pigmentary phenotypes, and risk of skin cancer in Caucasians.Int J Cancer. 2009 Aug 15;125(4):909-17. doi: 10.1002/ijc.24327. Int J Cancer. 2009. PMID: 19384953 Free PMC article. Clinical Trial.
-
Two independent retrotransposon insertions at the same site within the coding region of BTK.Hum Mutat. 2005 Mar;25(3):324-5. doi: 10.1002/humu.9321. Hum Mutat. 2005. PMID: 15712380
-
Skin Pigmentation Genetics for the Clinic.Dermatology. 2017;233(1):1-15. doi: 10.1159/000468538. Epub 2017 May 3. Dermatology. 2017. PMID: 28463841 Review.
-
L1 retrotransposons, cancer stem cells and oncogenesis.FEBS J. 2014 Jan;281(1):63-73. doi: 10.1111/febs.12601. Epub 2013 Nov 28. FEBS J. 2014. PMID: 24286172 Free PMC article. Review.
Cited by
-
Mobile element insertions affect human pigmentation and skin cancer risk.Nat Genet. 2024 Aug;56(8):1546-1547. doi: 10.1038/s41588-024-01849-w. Nat Genet. 2024. PMID: 39048793 No abstract available.
References
MeSH terms
Substances
Grants and funding
LinkOut - more resources
Full Text Sources
Miscellaneous