Starting DNA Synthesis: Initiation Processes during the Replication of Chromosomal DNA in Humans
- PMID: 38540419
- PMCID: PMC10969946
- DOI: 10.3390/genes15030360
Starting DNA Synthesis: Initiation Processes during the Replication of Chromosomal DNA in Humans
Abstract
The initiation reactions of DNA synthesis are central processes during human chromosomal DNA replication. They are separated into two main processes: the initiation events at replication origins, the start of the leading strand synthesis for each replicon, and the numerous initiation events taking place during lagging strand DNA synthesis. In addition, a third mechanism is the re-initiation of DNA synthesis after replication fork stalling, which takes place when DNA lesions hinder the progression of DNA synthesis. The initiation of leading strand synthesis at replication origins is regulated at multiple levels, from the origin recognition to the assembly and activation of replicative helicase, the Cdc45-MCM2-7-GINS (CMG) complex. In addition, the multiple interactions of the CMG complex with the eukaryotic replicative DNA polymerases, DNA polymerase α-primase, DNA polymerase δ and ε, at replication forks play pivotal roles in the mechanism of the initiation reactions of leading and lagging strand DNA synthesis. These interactions are also important for the initiation of signalling at unperturbed and stalled replication forks, "replication stress" events, via ATR (ATM-Rad 3-related protein kinase). These processes are essential for the accurate transfer of the cells' genetic information to their daughters. Thus, failures and dysfunctions in these processes give rise to genome instability causing genetic diseases, including cancer. In their influential review "Hallmarks of Cancer: New Dimensions", Hanahan and Weinberg (2022) therefore call genome instability a fundamental function in the development process of cancer cells. In recent years, the understanding of the initiation processes and mechanisms of human DNA replication has made substantial progress at all levels, which will be discussed in the review.
Keywords: ATR kinase; DNA damage signalling; DNA replication; Okazaki fragment; initiation reactions; origin of replication; replication fork; telomeres.
Conflict of interest statement
The authors declare no conflict of interest.
Figures
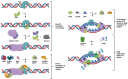
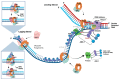
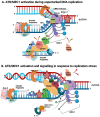
Similar articles
-
Eukaryotic DNA Replication Fork.Annu Rev Biochem. 2017 Jun 20;86:417-438. doi: 10.1146/annurev-biochem-061516-044709. Epub 2017 Mar 1. Annu Rev Biochem. 2017. PMID: 28301743 Free PMC article. Review.
-
Lagging Strand Initiation Processes in DNA Replication of Eukaryotes-Strings of Highly Coordinated Reactions Governed by Multiprotein Complexes.Genes (Basel). 2023 Apr 29;14(5):1012. doi: 10.3390/genes14051012. Genes (Basel). 2023. PMID: 37239371 Free PMC article. Review.
-
Termination of DNA replication forks: "Breaking up is hard to do".Nucleus. 2015;6(3):187-96. doi: 10.1080/19491034.2015.1035843. Epub 2015 Apr 2. Nucleus. 2015. PMID: 25835602 Free PMC article.
-
Quality control mechanisms exclude incorrect polymerases from the eukaryotic replication fork.Proc Natl Acad Sci U S A. 2017 Jan 24;114(4):675-680. doi: 10.1073/pnas.1619748114. Epub 2017 Jan 9. Proc Natl Acad Sci U S A. 2017. PMID: 28069954 Free PMC article.
-
The Eukaryotic CMG Helicase at the Replication Fork: Emerging Architecture Reveals an Unexpected Mechanism.Bioessays. 2018 Mar;40(3):10.1002/bies.201700208. doi: 10.1002/bies.201700208. Epub 2018 Feb 6. Bioessays. 2018. PMID: 29405332 Free PMC article. Review.
Cited by
-
Hypoxic reactivation of Kaposi's sarcoma associated herpesvirus.Cell Insight. 2024 Sep 7;3(6):100200. doi: 10.1016/j.cellin.2024.100200. eCollection 2024 Dec. Cell Insight. 2024. PMID: 39391006 Free PMC article. Review.
References
Publication types
MeSH terms
Substances
Grants and funding
LinkOut - more resources
Full Text Sources
Research Materials
Miscellaneous