Sound waves alter the viability of tobacco cells via changes in cytosolic calcium, membrane integrity, and cell wall composition
- PMID: 38466667
- PMCID: PMC10927088
- DOI: 10.1371/journal.pone.0299055
Sound waves alter the viability of tobacco cells via changes in cytosolic calcium, membrane integrity, and cell wall composition
Abstract
The effect of sound waves (SWs) on plant cells can be considered as important as other mechanical stimuli like touch, wind, rain, and gravity, causing certain responses associated with the downstream signaling pathways on the whole plant. The objective of the present study was to elucidate the response of suspension-cultured tobacco cells (Nicotiana tabacum L. cv Burley 21) to SW at different intensities. The sinusoidal SW (1,000 Hz) was produced through a signal generator, amplified, and beamed to the one layer floating tobacco cells inside a soundproof chamber at intensities of 60, 75, and 90 dB at the plate level for 15, 30, 45, and 60 min. Calibration of the applied SW intensities, accuracy, and uniformity of SW was performed by a sound level meter, and the cells were treated. The effect of SW on tobacco cells was monitored by quantitation of cytosolic calcium, redox status, membrane integrity, wall components, and the activity of wall modifying enzymes. Cytosolic calcium ions increased as a function of sound intensity with a maximum level of 90 dB. Exposure to 90 dB was also accompanied by a significant increase of H2O2 and membrane lipid peroxidation rate but the reduction of total antioxidant and radical scavenging capacities. The increase of wall rigidity in these cells was attributed to an increase in wall-bound phenolic acids and lignin and the activities of phenylalanine ammonia-lyase and covalently bound peroxidase. In comparison, in 60- and 75 dB, radical scavenging capacity increased, and the activity of wall stiffening enzymes reduced, but cell viability showed no changes. The outcome of the current study reveals that the impact of SW on plant cells is started by an increase in cytosolic calcium. However, upon calcium signaling, downstream events, including alteration of H2O2 and cell redox status and the activities of wall modifying enzymes, determined the extent of SW effects on tobacco cells.
Copyright: © 2024 Sardari et al. This is an open access article distributed under the terms of the Creative Commons Attribution License, which permits unrestricted use, distribution, and reproduction in any medium, provided the original author and source are credited.
Conflict of interest statement
The authors have declared that no competing interests exist.
Figures
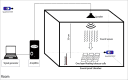
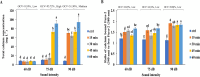
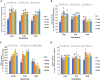
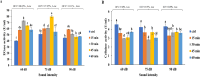
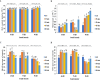
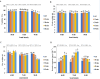
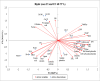
Similar articles
-
Proteomic analysis reveals a novel set of cell wall proteins in a transformed tobacco cell culture that synthesises secondary walls as determined by biochemical and morphological parameters.Planta. 2001 Feb;212(3):404-15. doi: 10.1007/s004250000407. Planta. 2001. PMID: 11289605
-
Molecular and biochemical modifications of suspension-cultured tobacco cell walls after exposure to alternative gravity.Plant Physiol Biochem. 2022 Apr 1;176:1-7. doi: 10.1016/j.plaphy.2022.02.012. Epub 2022 Feb 14. Plant Physiol Biochem. 2022. PMID: 35180456
-
Mechanism of peroxidase actions for salicylic acid-induced generation of active oxygen species and an increase in cytosolic calcium in tobacco cell suspension culture.J Exp Bot. 2000 Apr;51(345):685-93. J Exp Bot. 2000. PMID: 10938860
-
Calcium signaling in plant cells in altered gravity.Adv Space Res. 2003;32(8):1621-30. doi: 10.1016/S0273-1177(03)90403-0. Adv Space Res. 2003. PMID: 15002419 Review.
-
Ionic signaling in plant responses to gravity and touch.J Plant Growth Regul. 2002 Jun;21(2):71-88. doi: 10.1007/s003440010049. Epub 2002 May 23. J Plant Growth Regul. 2002. PMID: 12016507 Review.
References
MeSH terms
Substances
Grants and funding
LinkOut - more resources
Full Text Sources
Miscellaneous