Multiple Photolyases Protect the Marine Cyanobacterium Synechococcus from Ultraviolet Radiation
- PMID: 35856560
- PMCID: PMC9426592
- DOI: 10.1128/mbio.01511-22
Multiple Photolyases Protect the Marine Cyanobacterium Synechococcus from Ultraviolet Radiation
Abstract
Marine cyanobacteria depend on light for photosynthesis, restricting their growth to the photic zone. The upper part of this layer is exposed to strong UV radiation (UVR), a DNA mutagen that can harm these microorganisms. To thrive in UVR-rich waters, marine cyanobacteria employ photoprotection strategies that are still not well defined. Among these are photolyases, light-activated enzymes that repair DNA dimers generated by UVR. Our analysis of genomes of 81 strains of Synechococcus, Cyanobium, and Prochlorococcus isolated from the world's oceans shows that they possess up to five genes encoding different members of the photolyase/cryptochrome family, including a photolyase with a novel domain arrangement encoded by either one or two separate genes. We disrupted the putative photolyase-encoding genes in Synechococcus sp. strain RS9916 and discovered that each gene contributes to the overall capacity of this organism to survive UVR. Additionally, each conferred increased survival after UVR exposure when transformed into Escherichia coli lacking its photolyase and SOS response. Our results provide the first evidence that this large set of photolyases endows Synechococcus with UVR resistance that is far superior to that of E. coli, but that, unlike for E. coli, these photolyases provide Synechococcus with the vast majority of its UVR tolerance. IMPORTANCE Cells use DNA photolyases to protect their DNA from the damaging effects of UV radiation. Marine cyanobacteria possess many genes that appear to encode photolyases, but the function of the proteins encoded by these genes is unclear. The study uses comparative genomics and molecular genetic approaches to describe and characterize the roles of these proteins in DNA damage repair in the marine cyanobacterium Synechococcus. This study identifies the important role of DNA photolyases in DNA repair for these cells and describes a previously undescribed structural class of DNA of these enzymes.
Keywords: DNA photolyase; Synechococcus; UV light; cyanobacteria; marine microbiology.
Conflict of interest statement
The authors declare no conflict of interest.
Figures
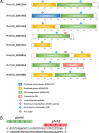
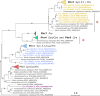
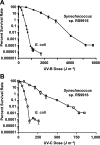
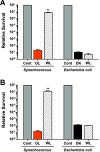
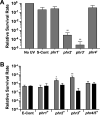
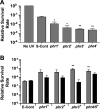
Similar articles
-
Genetic and phylogenetic characterization of the type II cyclobutane pyrimidine dimer photolyases encoded by Leporipoxviruses.Virology. 2003 Oct 10;315(1):10-9. doi: 10.1016/s0042-6822(03)00512-9. Virology. 2003. PMID: 14592755
-
A putative bifunctional CPD/ (6-4) photolyase from the cyanobacteria Synechococcus sp. PCC 7335 is encoded by a UV-B inducible operon: New insights into the evolution of photolyases.Front Microbiol. 2022 Oct 28;13:981788. doi: 10.3389/fmicb.2022.981788. eCollection 2022. Front Microbiol. 2022. PMID: 36386616 Free PMC article.
-
Bacterial cryptochrome and photolyase: characterization of two photolyase-like genes of Synechocystis sp. PCC6803.Nucleic Acids Res. 2000 Jun 15;28(12):2353-62. doi: 10.1093/nar/28.12.2353. Nucleic Acids Res. 2000. PMID: 10871367 Free PMC article.
-
Photolyase Production and Current Applications: A Review.Molecules. 2022 Sep 15;27(18):5998. doi: 10.3390/molecules27185998. Molecules. 2022. PMID: 36144740 Free PMC article. Review.
-
Photolyases: capturing the light to battle skin cancer.Future Oncol. 2006 Apr;2(2):191-9. doi: 10.2217/14796694.2.2.191. Future Oncol. 2006. PMID: 16563088 Review.
Cited by
-
Different photosynthetic responses of haploid and diploid Emiliania huxleyi (Prymnesiophyceae) to high light and ultraviolet radiation.Bioresour Bioprocess. 2023 Jul 14;10(1):40. doi: 10.1186/s40643-023-00660-5. Bioresour Bioprocess. 2023. PMID: 38647570 Free PMC article.
-
Glutamine coated titanium for synergistic sonodynamic and photothermal on tumor therapy upon targeted delivery.Front Bioeng Biotechnol. 2023 Apr 10;11:1139426. doi: 10.3389/fbioe.2023.1139426. eCollection 2023. Front Bioeng Biotechnol. 2023. PMID: 37101748 Free PMC article.
-
An unexpected role for leucyl aminopeptidase in UV tolerance revealed by a genome-wide fitness assessment in a model cyanobacterium.Proc Natl Acad Sci U S A. 2022 Nov 8;119(45):e2211789119. doi: 10.1073/pnas.2211789119. Epub 2022 Nov 2. Proc Natl Acad Sci U S A. 2022. PMID: 36322730 Free PMC article.
References
-
- Llabres M, Agusti S. 2006. Picophytoplankton cell death induced by UV radiation: evidence for oceanic Atlantic communities. Limnol Oceanogr 51:21–29. doi:10.4319/lo.2006.51.1.0021. - DOI
-
- Llabres M, Agusti S, Alonso-Laita P, Herndl GJ. 2010. Synechococcus and Prochlorococcus cell death induced by UV radiation and the penetration of lethal UVR in the Mediterranean Sea. Mar Ecol Prog Ser 399:27–37. doi:10.3354/meps08332. - DOI
Publication types
MeSH terms
Substances
Grants and funding
LinkOut - more resources
Full Text Sources