Regulation of Rostral Nucleus of the Solitary Tract Responses to Afferent Input by A-type K+ Current
- PMID: 35659639
- PMCID: PMC9253083
- DOI: 10.1016/j.neuroscience.2022.05.036
Regulation of Rostral Nucleus of the Solitary Tract Responses to Afferent Input by A-type K+ Current
Abstract
Responses in the rostral (gustatory) nucleus of the solitary tract (rNST) are modified by synaptic interactions within the nucleus and the constitutive membrane properties of the neurons themselves. The potassium current IA is one potential source of modulation. In the caudal NST, projection neurons with IA show lower fidelity to afferent stimulation compared to cells without. We explored the role of an A-type K+ current (IA) in modulating the response to afferent stimulation and GABA-mediated inhibition in the rNST using whole cell patch clamp recording in transgenic mice that expressed channelrhodopsin (ChR2 H134R) in GABAergic neurons. The presence of IA was determined in current clamp and the response to electrical stimulation of afferent fibers in the solitary tract was assessed before and after treatment with the specific Kv4 channel blocker AmmTX3. Blocking IA significantly increased the response to afferent stimulation by 53%. Using dynamic clamp to create a synthetic IA conductance, we demonstrated a significant 14% decrease in responsiveness to afferent stimulation in cells lacking IA. Because IA reduced excitability and is hyperpolarization-sensitive, we examined whether IA contributed to the inhibition resulting from optogenetic release of GABA. Although blocking IA decreased the percent suppression induced by GABA, this effect was attributable to the increased responsiveness resulting from AmmTX3, not to a change in the absolute magnitude of suppression. We conclude that rNST responses to afferent input are regulated independently by IA and GABA.
Keywords: dynamic clamp; inhibition; patch clamp; taste.
Copyright © 2022 IBRO. Published by Elsevier Ltd. All rights reserved.
Figures
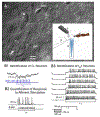
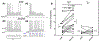
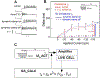
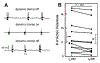
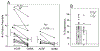
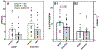
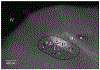
Similar articles
-
Kv4 channel expression and kinetics in GABAergic and non-GABAergic rNST neurons.J Neurophysiol. 2020 Dec 1;124(6):1727-1742. doi: 10.1152/jn.00396.2020. Epub 2020 Sep 30. J Neurophysiol. 2020. PMID: 32997557 Free PMC article.
-
Inhibitory modulation of optogenetically identified neuron subtypes in the rostral solitary nucleus.J Neurophysiol. 2016 Aug 1;116(2):391-403. doi: 10.1152/jn.00168.2016. Epub 2016 May 4. J Neurophysiol. 2016. PMID: 27146980 Free PMC article.
-
Synaptic interactions due to convergent input from gustatory afferent fibers in the rostral nucleus of the solitary tract.J Neurophysiol. 1996 Nov;76(5):2919-27. doi: 10.1152/jn.1996.76.5.2919. J Neurophysiol. 1996. PMID: 8930244
-
Neural circuits for taste. Excitation, inhibition, and synaptic plasticity in the rostral gustatory zone of the nucleus of the solitary tract.Ann N Y Acad Sci. 1998 Nov 30;855:467-74. doi: 10.1111/j.1749-6632.1998.tb10607.x. Ann N Y Acad Sci. 1998. PMID: 9929640 Review.
-
Neurotransmitter and neuromodulator activity in the gustatory zone of the nucleus tractus solitarius.Chem Senses. 1996 Jun;21(3):377-85. doi: 10.1093/chemse/21.3.377. Chem Senses. 1996. PMID: 8670717 Review.
References
-
- Bailey TW, Hermes SM, Andresen MC, and Aicher SA. Cranial visceral afferent pathways through the nucleus of the solitary tract to caudal ventrolateral medulla or paraventricular hypothalamus: target-specific synaptic reliability and convergence patterns. J Neurosci 26: 11893–11902, 2006. - PMC - PubMed
-
- Beckman ME, and Whitehead MC. Intramedullary connections of the rostral nucleus of the solitary tract in the hamster. Brain Res 557: 265–279, 1991. - PubMed
Publication types
MeSH terms
Substances
Grants and funding
LinkOut - more resources
Full Text Sources
Molecular Biology Databases