Shaping human telomeres: from shelterin and CST complexes to telomeric chromatin organization
- PMID: 33564154
- PMCID: PMC8221230
- DOI: 10.1038/s41580-021-00328-y
Shaping human telomeres: from shelterin and CST complexes to telomeric chromatin organization
Erratum in
-
Publisher Correction: Shaping human telomeres: from shelterin and CST complexes to telomeric chromatin organization.Nat Rev Mol Cell Biol. 2021 Apr;22(4):299. doi: 10.1038/s41580-021-00353-x. Nat Rev Mol Cell Biol. 2021. PMID: 33608692 No abstract available.
Abstract
The regulation of telomere length in mammals is crucial for chromosome end-capping and thus for maintaining genome stability and cellular lifespan. This process requires coordination between telomeric protein complexes and the ribonucleoprotein telomerase, which extends the telomeric DNA. Telomeric proteins modulate telomere architecture, recruit telomerase to accessible telomeres and orchestrate the conversion of the newly synthesized telomeric single-stranded DNA tail into double-stranded DNA. Dysfunctional telomere maintenance leads to telomere shortening, which causes human diseases including bone marrow failure, premature ageing and cancer. Recent studies provide new insights into telomerase-related interactions (the 'telomere replisome') and reveal new challenges for future telomere structural biology endeavours owing to the dynamic nature of telomere architecture and the great number of structures that telomeres form. In this Review, we discuss recently determined structures of the shelterin and CTC1-STN1-TEN1 (CST) complexes, how they may participate in the regulation of telomere replication and chromosome end-capping, and how disease-causing mutations in their encoding genes may affect specific functions. Major outstanding questions in the field include how all of the telomere components assemble relative to each other and how the switching between different telomere structures is achieved.
Conflict of interest statement
Competing interests
T.R.C. is on the board of directors of Merck and a consultant for STORM Therapeutics and Eikon Therapeutics.
Figures
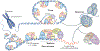
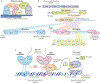
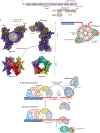
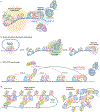
Similar articles
-
Structural biology of telomeres and telomerase.Cell Mol Life Sci. 2020 Jan;77(1):61-79. doi: 10.1007/s00018-019-03369-x. Epub 2019 Nov 14. Cell Mol Life Sci. 2020. PMID: 31728577 Free PMC article. Review.
-
DNA-Directed Polymerase Subunits Play a Vital Role in Human Telomeric Overhang Processing.Mol Cancer Res. 2015 Mar;13(3):402-10. doi: 10.1158/1541-7786.MCR-14-0381. Epub 2014 Dec 17. Mol Cancer Res. 2015. PMID: 25519149 Free PMC article.
-
POT1 recruits and regulates CST-Polα/primase at human telomeres.Cell. 2024 Jul 11;187(14):3638-3651.e18. doi: 10.1016/j.cell.2024.05.002. Epub 2024 Jun 4. Cell. 2024. PMID: 38838667
-
The fission yeast Stn1-Ten1 complex limits telomerase activity via its SUMO-interacting motif and promotes telomeres replication.Sci Adv. 2018 May 16;4(5):eaar2740. doi: 10.1126/sciadv.aar2740. eCollection 2018 May. Sci Adv. 2018. PMID: 29774234 Free PMC article.
-
CST-Polα/Primase: the second telomere maintenance machine.Genes Dev. 2023 Jul 1;37(13-14):555-569. doi: 10.1101/gad.350479.123. Epub 2023 Jul 26. Genes Dev. 2023. PMID: 37495394 Free PMC article. Review.
Cited by
-
3D-Q-FISH/Telomere/TRF2 Nanotechnology Identifies a Progressively Disturbed Telomere/Shelterin/Lamin AC Complex as the Common Pathogenic, Molecular/Spatial Denominator of Classical Hodgkin Lymphoma.Cells. 2024 Oct 23;13(21):1748. doi: 10.3390/cells13211748. Cells. 2024. PMID: 39513855 Free PMC article. Review.
-
Dysfunction of Telomeric Cdc13-Stn1-Ten1 Simultaneously Activates DNA Damage and Spindle Checkpoints.Cells. 2024 Sep 25;13(19):1605. doi: 10.3390/cells13191605. Cells. 2024. PMID: 39404369 Free PMC article.
-
Inherited Telomere Biology Disorders: Pathophysiology, Clinical Presentation, Diagnostics, and Treatment.Transfus Med Hemother. 2024 Jul 30;51(5):292-309. doi: 10.1159/000540109. eCollection 2024 Oct. Transfus Med Hemother. 2024. PMID: 39371255 Free PMC article. Review.
-
Searching for Beauty and Health: Aging in Women, Nutrition, and the Secret in Telomeres.Nutrients. 2024 Sep 15;16(18):3111. doi: 10.3390/nu16183111. Nutrients. 2024. PMID: 39339711 Free PMC article. Review.
-
Telomeres and telomerase in Sarcoma disease and therapy.Int J Med Sci. 2024 Aug 6;21(11):2065-2080. doi: 10.7150/ijms.97485. eCollection 2024. Int J Med Sci. 2024. PMID: 39239547 Free PMC article. Review.
References
Publication types
MeSH terms
Substances
Grants and funding
LinkOut - more resources
Full Text Sources
Other Literature Sources