Microbial peptidyl-prolyl cis/trans isomerases (PPIases): virulence factors and potential alternative drug targets
- PMID: 25184565
- PMCID: PMC4187684
- DOI: 10.1128/MMBR.00015-14
Microbial peptidyl-prolyl cis/trans isomerases (PPIases): virulence factors and potential alternative drug targets
Abstract
Initially discovered in the context of immunomodulation, peptidyl-prolyl cis/trans isomerases (PPIases) were soon identified as enzymes catalyzing the rate-limiting protein folding step at peptidyl bonds preceding proline residues. Intense searches revealed that PPIases are a superfamily of proteins consisting of three structurally distinguishable families with representatives in every described species of prokaryote and eukaryote and, recently, even in some giant viruses. Despite the clear-cut enzymatic activity and ubiquitous distribution of PPIases, reports on solely PPIase-dependent biological roles remain scarce. Nevertheless, they have been found to be involved in a plethora of biological processes, such as gene expression, signal transduction, protein secretion, development, and tissue regeneration, underscoring their general importance. Hence, it is not surprising that PPIases have also been identified as virulence-associated proteins. The extent of contribution to virulence is highly variable and dependent on the pleiotropic roles of a single PPIase in the respective pathogen. The main objective of this review is to discuss this variety in virulence-related bacterial and protozoan PPIases as well as the involvement of host PPIases in infectious processes. Moreover, a special focus is given to Legionella pneumophila macrophage infectivity potentiator (Mip) and Mip-like PPIases of other pathogens, as the best-characterized virulence-related representatives of this family. Finally, the potential of PPIases as alternative drug targets and first tangible results are highlighted.
Copyright © 2014, American Society for Microbiology. All Rights Reserved.
Figures
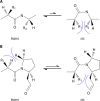
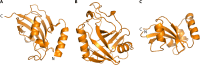
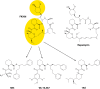
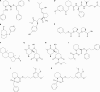
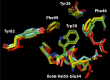
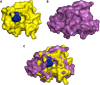
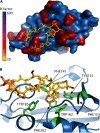
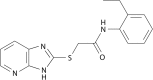
Similar articles
-
Vaccine potential of bacterial macrophage infectivity potentiator (MIP)-like peptidyl prolyl cis/trans isomerase (PPIase) proteins.Expert Rev Vaccines. 2015;14(12):1633-49. doi: 10.1586/14760584.2015.1095638. Epub 2015 Oct 15. Expert Rev Vaccines. 2015. PMID: 26468663 Review.
-
Peptidylprolyl cis-trans isomerases of Legionella pneumophila: virulence, moonlighting and novel therapeutic targets.Biochem Soc Trans. 2014 Dec;42(6):1728-33. doi: 10.1042/BST20140202. Biochem Soc Trans. 2014. PMID: 25399597 Review.
-
Mip protein of Legionella pneumophila exhibits peptidyl-prolyl-cis/trans isomerase (PPlase) activity.Mol Microbiol. 1992 May;6(10):1375-83. doi: 10.1111/j.1365-2958.1992.tb00858.x. Mol Microbiol. 1992. PMID: 1379319
-
FKBPs in bacterial infections.Biochim Biophys Acta. 2015 Oct;1850(10):2096-102. doi: 10.1016/j.bbagen.2014.12.018. Epub 2014 Dec 19. Biochim Biophys Acta. 2015. PMID: 25529296 Review.
-
An Intracellular Peptidyl-Prolyl cis/trans Isomerase Is Required for Folding and Activity of the Staphylococcus aureus Secreted Virulence Factor Nuclease.J Bacteriol. 2016 Dec 13;199(1):e00453-16. doi: 10.1128/JB.00453-16. Print 2017 Jan 1. J Bacteriol. 2016. PMID: 27795319 Free PMC article.
Cited by
-
Cyclic Isothiocyanate Goitrin Impairs Lotus japonicus Nodulation, Affects the Proteomes of Nodules and Free Mesorhizobium loti, and Induces the Formation of Caffeic Acid Derivatives in Bacterial Cultures.Plants (Basel). 2024 Oct 16;13(20):2897. doi: 10.3390/plants13202897. Plants (Basel). 2024. PMID: 39458844 Free PMC article.
-
Dosage constraint of the ribosome-associated molecular chaperone drives the evolution and fates of its duplicates in bacteria.mBio. 2024 Nov 13;15(11):e0199424. doi: 10.1128/mbio.01994-24. Epub 2024 Oct 7. mBio. 2024. PMID: 39373534 Free PMC article.
-
High Affinity Inhibitors of the Macrophage Infectivity Potentiator Protein from Trypanosoma cruzi, Burkholderia pseudomallei, and Legionella pneumophila─A Comparison.ACS Infect Dis. 2024 Oct 11;10(10):3681-3691. doi: 10.1021/acsinfecdis.4c00553. Epub 2024 Oct 2. ACS Infect Dis. 2024. PMID: 39357850
-
Insights into Peptidyl-Prolyl cis-trans Isomerases from Clinically Important Protozoans: From Structure to Potential Biotechnological Applications.Pathogens. 2024 Jul 31;13(8):644. doi: 10.3390/pathogens13080644. Pathogens. 2024. PMID: 39204244 Free PMC article. Review.
-
Proteomes of native and non-native symbionts reveal responses underpinning host-symbiont specificity in the cnidarian-dinoflagellate symbiosis.ISME J. 2024 Jan 8;18(1):wrae122. doi: 10.1093/ismejo/wrae122. ISME J. 2024. PMID: 38988135 Free PMC article.
References
Publication types
MeSH terms
Substances
LinkOut - more resources
Full Text Sources
Other Literature Sources
Molecular Biology Databases