Garrod's fourth inborn error of metabolism solved by the identification of mutations causing pentosuria
- PMID: 22042873
- PMCID: PMC3215002
- DOI: 10.1073/pnas.1115888108
Garrod's fourth inborn error of metabolism solved by the identification of mutations causing pentosuria
Abstract
Pentosuria is one of four conditions hypothesized by Archibald Garrod in 1908 to be inborn errors of metabolism. Mutations responsible for the other three conditions (albinism, alkaptonuria, and cystinuria) have been identified, but the mutations responsible for pentosuria remained unknown. Pentosuria, which affects almost exclusively individuals of Ashkenazi Jewish ancestry, is characterized by high levels of the pentose sugar L-xylulose in blood and urine and deficiency of the enzyme L-xylulose reductase. The condition is autosomal-recessive and completely clinically benign, but in the early and mid-20th century attracted attention because it was often confused with diabetes mellitus and inappropriately treated with insulin. Persons with pentosuria were identified from records of Margaret Lasker, who studied the condition in the 1930s to 1960s. In the DCXR gene encoding L-xylulose reductase, we identified two mutations, DCXR c.583ΔC and DCXR c.52(+1)G > A, each predicted to lead to loss of enzyme activity. Of nine unrelated living pentosuric subjects, six were homozygous for DCXR c.583ΔC, one was homozygous for DCXR c.52(+1)G > A, and two were compound heterozygous for the two mutant alleles. L-xylulose reductase was not detectable in protein lysates from subjects' cells and high levels of xylulose were detected in their sera, confirming the relationship between the DCXR genotypes and the pentosuric phenotype. The combined frequency of the two mutant DCXR alleles in 1,067 Ashkenazi Jewish controls was 0.0173, suggesting a pentosuria frequency of approximately one in 3,300 in this population. Haplotype analysis indicated that the DCXR c.52(+1)G > A mutation arose more recently than the DCXR c.583ΔC mutation.
Conflict of interest statement
The authors declare no conflict of interest.
Figures
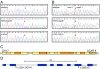
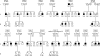
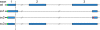
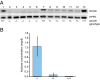
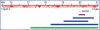
Similar articles
-
Dicarbonyl/l-xylulose reductase (DCXR): The multifunctional pentosuria enzyme.Int J Biochem Cell Biol. 2013 Nov;45(11):2563-7. doi: 10.1016/j.biocel.2013.08.010. Epub 2013 Aug 27. Int J Biochem Cell Biol. 2013. PMID: 23988570 Review.
-
Milestones in treatments for inborn errors of metabolism: Reflections on Where chemistry and medicine meet.Am J Med Genet A. 2021 Nov;185(11):3350-3358. doi: 10.1002/ajmg.a.62385. Epub 2021 Jun 24. Am J Med Genet A. 2021. PMID: 34165242 Review.
-
Human L-xylulose reductase variation: family and population studies.Ann Hum Genet. 1985 Jul;49(3):227-35. doi: 10.1111/j.1469-1809.1985.tb01696.x. Ann Hum Genet. 1985. PMID: 4073836
-
Sir Archibald Garrod's Inborn Errors of Metabolism. IV. Pentosuria.Am J Hum Genet. 1958 Dec;10(4):385-97. Am J Hum Genet. 1958. PMID: 13606116 Free PMC article. No abstract available.
-
Human DCXR - another 'moonlighting protein' involved in sugar metabolism, carbonyl detoxification, cell adhesion and male fertility?Biol Rev Camb Philos Soc. 2015 Feb;90(1):254-78. doi: 10.1111/brv.12108. Epub 2014 Apr 10. Biol Rev Camb Philos Soc. 2015. PMID: 24720935 Review.
Cited by
-
A novel L-xylulose reductase essential for L-arabinose catabolism in Trichoderma reesei.Biochemistry. 2013 Apr 9;52(14):2453-60. doi: 10.1021/bi301583u. Epub 2013 Mar 29. Biochemistry. 2013. PMID: 23506391 Free PMC article.
-
Evolutionary molecular medicine.J Mol Med (Berl). 2012 May;90(5):509-22. doi: 10.1007/s00109-012-0889-9. Epub 2012 Apr 29. J Mol Med (Berl). 2012. PMID: 22544168 Free PMC article. Review.
References
-
- Garrod AE. The Croonian lectures on inborn errors of metabolism, lecture IV. Lancet. 1908;2:214–220.
-
- Bearn AG. Archibald Garrod and the Individuality of Man. Oxford: Clarendon Press; 1993.
-
- De Vries H. The law of segregation of hybrids. Berichte der Deutschen Botanischem Gesellsshaft. 1900;18:83–90. German.
-
- Harper PS. A Short History of Medical Genetics. Oxford: Oxford University Press; 2008.
-
- Scriver CR. Garrod's Croonian Lectures (1908) and the charter ‘Inborn Errors of Metabolism’: Albinism, alkaptonuria, cystinuria, and pentosuria at age 100 in 2008. J Inherit Metab Dis. 2008;31:580–598. - PubMed
Publication types
MeSH terms
Substances
Supplementary concepts
Grants and funding
LinkOut - more resources
Full Text Sources
Other Literature Sources
Medical
Molecular Biology Databases
Miscellaneous